Curing Diabetes: A Quest For The Holy Grail
Diabetes has become an epidemic, condemning over 537 million people worldwide to taking medication for life. The total number of diabetics is expected to climb to 643 million by 2030 and 783 million by 2045 (Kumar et al., 2023). Diabetes mellitus is a chronic metabolic disorder characterized by the body’s inability to maintain normal blood glucose levels. Glucose is a sugar that serves as the primary fuel for cells in the body and is converted to either energy or fat for long-term storage. However, glucose relies on insulin to enter cells. Insulin is a key hormone produced by the pancreas, which once bound to cells allows glucose influx, hence reducing high blood glucose levels (Satoh, 2014). Diabetes occurs when the pancreas either fails to produce insulin (type 1 diabetes, T1D) or the body is unable to properly use the insulin produced by the pancreas (type 2 diabetes, T2D). To better understand this, consider insulin as a key that, when fitted into the proper door lock, unlocks the cell's ability to absorb glucose. Patients with T1D are unable to produce insulin and hence lack the key that allows glucose to enter. Type 2 diabetics, on the other hand, may still produce insulin and so have the key, but their cells lack the ability to recognize and respond to it, much like attempting to unlock a door with the wrong key (Diabetes, 2023). Glucose is mainly produced during digestion when carbohydrates from food are broken down. As a result, healthy individual's pancreas produces more insulin after a meal, allowing glucose to enter cells, thereby lowering blood sugar levels. If, on the other hand, blood sugar levels drop, such as after an extended fast, the pancreas produces the hormone glucagon. This hormone induces the breakdown of the energy-storing polysaccharide glycogen into glucose, hence restoring blood glucose levels (Bell, 2023). This homeostatic equilibrium is crucial for delivering appropriate energy to cells while minimizing the damage caused by sustained high blood sugar levels. This equilibrium, however, is lost in diabetic individuals.
The hallmark of diabetes, whether type 1 or type 2, is hyperglycemia, the medical term for a high blood sugar (glucose) level. Symptoms of hyperglycemia include increased thirst (polydipsia), hunger (polyphagia), and urination (polyuria) as well as fatigue, unexpected weight loss, mood swings, and vision loss (American Diabetes Association, 2009; Baeshen et al., 2014). Long-term hyperglycemia often leads to various diabetic microvascular and macrovascular problems that damage important organs supplied by the circulatory system and are a major cause of diabetes-related morbidity and death. Diabetes-related vascular damage often results in nephropathy (deterioration of kidney function), retinopathy (growth of abnormal blood vessels in the retina), neuropathy (damage to the peripheral nervous system) and atherosclerosis (accumulation of lipids and cholesterol inside and around the walls of arteries) which predispose diabetic patients to diseases such as heart attack, stroke, kidney failure, and loss of vision. Nerve injuries and poor circulation can lead to foot ulcers and, in the worst-case scenario, amputations (Fowler, 2008; Jensen & Deckert, 1992).
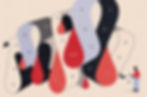
Two Sides of the Same Coin
Diabetes is often categorized into two types: autoimmune (T1D) and non-autoimmune (T2D), which despite being two manifestations of the same disease, diverge in multiple aspects. While T2D is typically a milder form of disease and in some instances is reversible with appropriate lifestyle changes and treatment regimens; T1D is incurable and requires insulin replacement and lifelong therapy (Biggers, 2022; Todd, 2010). Moreover, T1D arises in adolescence and is assumed to be driven by an autoimmune response, which occurs when the immune system mistakenly identifies the body's healthy cells as foreign invaders. As a result, the immune system attacks and destroys the insulin-producing beta cells in the pancreas, hindering it from producing insulin. While it is still unknown why the immune system occasionally attacks cells in the body, T1D can be attributed to certain genetic and environmental variables, such as viral exposure (Bluestone et al., 2010; Todd, 2010). By contrast, T2D develops gradually and is connected to lifestyle factors such as sedentary behavior and obesity, and it is most commonly diagnosed in adults, accounting for more than 90% of diabetes cases globally (Reed et al., 2021).
Because the pathophysiology of both forms of diabetes differs, so does the management and treatment. Since blood sugar levels can rise rapidly in T1D, regular insulin administration is crucial. Patients with T1D must routinely check their blood sugar levels to avoid hypoglycemia (dangerously low blood sugar levels) or hyperglycemia (extremely high blood sugar levels). Both conditions can lead to diabetic coma, a life-threatening condition that requires immediate medical attention (Illa & Sańchez, 1975). Many insulin-treated individuals are prone to hypoglycemia due to a mismatch between nutritional intake and insulin administration. Hypoglycemia must be addressed rapidly with glucose or glucagon administration, otherwise brain damage may ensue (McCall, 2012). On the other hand, T2D is often considerably simpler to treat and the bulk of treatment regimens include tracking blood sugar levels and adopting lifestyle changes, particularly integrating healthier eating habits, increasing physical activity, and managing weight. However, in some situations, lifestyle adjustments are insufficient to keep T2D under control, and medication therapy or even insulin is required. Metformin, which reduces blood sugar levels and enhances the body's reaction to insulin, is the first-line therapy for most patients with T2D (Aroda & Ratner, 2018).
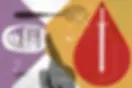
Diabetes is a complex disease the management of which goes far beyond glucose monitoring, since numerous factors can hinder reliable blood glucose control. Diet, physical activity, medication, stress, sickness, hormonal fluctuations, and dehydration are all examples of contributing factors for poor disease management (American Diabetes Association, 2017). Diabetes management is therefore a complicated, demanding, and sometimes puzzling set of self-care instructions, akin to solving a difficult, multi-variable arithmetic equation on a daily basis, for which there is no universal solution. Daily carbohydrate monitoring, estimating insulin dosage based on the insulin-to-carbohydrate ratio, adapting insulin amount during exercise, forecasting and mitigating risks, such as drinking or eating when glucose levels start to drop, are all part of this process. All of this renders disease self-management, i.e., the everyday tasks or actions a person must undertake to maintain blood sugar levels and mitigate the negative effects of the disease, extremely difficult (Wardian, 2017). Since the discovery of insulin, a century ago, the pharmaceutical industry has strived to make diabetes control easier, less invasive, and more effective with the aim of improving disease management and ultimately chasing diabetes’ holy grail: a cure.
Artificial Pancreas: The Jigsaw Piece for Managing Type 1 Diabetes
In T1D, blood glucose management is achieved by either numerous daily insulin injections or continuous subcutaneous insulin infusion using an insulin pump, a tiny computerized device that distributes insulin through a small cannula implanted under the patient's skin (Dansinger, 2022). However, T1D disease treatment is also based on regular glucose testing. A blood glucose meter (or glucometer) is a portable medical instrument that monitors blood glucose levels by pricking a fingertip and applying a drop of blood to a test strip (Harris, 2023). Noteworthy, the use of needle-free blood glucose meters such as flash glucose meters (FGM) or continuous glucose meters (CGM) has surged significantly over the last decade. Both are sensors that are implanted under the skin, usually in the abdomen or arm, and last a few weeks before needing to be replaced. Traditional blood glucose meters are still more accurate as they detect glucose in whole blood, but only provide static data on glucose levels and most of them still require a finger prick to collect blood, which is uncomfortable and sometimes painful (Hayes, 2020). Unlike traditional blood glucose meters, CGM and FGM sensors measure interstitial glucose, or glucose contained in the fluid between cells. While glucose levels are manually measured with a FGM by scanning the device over the sensor, a GCM routinely and automatically measures glucose levels and relays them to a device or smartphone through Bluetooth. In 2014, Abbott launched the Freestyle Libre in Europe, the first FDA-approved FGM and a breakthrough step in needle-free glucose monitoring (Brown, 2018). CGM technology, on the other hand, offers additional benefits by automatically capturing dynamic changes in glucose levels over time, hypo- and hyperglycemic episodes, trends, and patterns, allowing clinicians to direct therapy more successfully (Porch, 2023). However, low precision remains the primary drawback of this technology, which is why some devices require regular manual calibrations with manual blood glucose measurements (Continuous Glucose Monitoring, 2017).
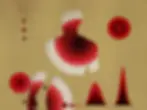
While CGMs are among the most important developments in recent biomedical tech the majority of patients do not achieve optimal glycemic control with current therapies, and new technologies offer new hope in alleviating the burden of T1D (Alcántara-Aragón, 2019). The automated artificial pancreas has emerged as the missing piece in the puzzle of glucose control for T1D patients. While the concept of an artificial pancreas, also known as a closed-loop system, has been around since the late 1970s, technological advancements have only lately made it a viable option for T1D patients (Albisser et al., 1974). A single system composed of a continuous CGM, an insulin pump, and an algorithm checks blood glucose levels and automatically delivers insulin, much like a normal pancreas would. In 2016, the US Food and Drug Administration gave its approval to the first artificial pancreas system, the hybrid closed-loop Medtronics MiniMed 670G system (Caccomo, 2018). This system combines a CGM to assess blood sugar levels every five minutes and automatically distributes the necessary dose of basal insulin, a long-acting insulin, through a separate pump. It is called hybrid because it still requires considerable user input.
Most available systems on the market can only anticipate outcomes when the user is not eating or exercising, and automatically switch to manual mode if blood sugar levels are abnormally too high or too low. One of the most difficult problems in building a fully automated artificial pancreas is that current insulin formulations cannot respond rapidly enough to significant variations in blood sugar, such as those following a meal (Fernández, 2019). Last year, however, a research team at Massachusetts General Hospital, funded by the National Institutes of Health (NIH), designed an automated insulin delivery system that employs next-generation technology to autonomously deliver insulin and so requires less human input than commercially available devices. This electronic device, known as a bionic pancreas, has been more successful than standard therapy in maintaining blood sugar levels within the normal range in individuals with T1D. Remarkably, the only information required to set up the device is the user's body weight and an estimate of carbohydrate intake. Otherwise, insulin administration is fully automatic (Voss, 2022).
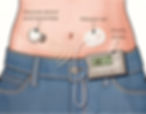
Reaching the Biological Cure: From Islet Transplantation to Cell Replacement
Cell therapy, albeit still in its infancy, is one of the most promising treatments for T1D. Replacing lost insulin-producing cells can potentially restore normal insulin production and cure patients. Full pancreatic function has been successfully restored in a small subset of patients by transplantation of the entire pancreas or pancreatic islets, also known as islets of Langerhans. These are regions of the pancreas that contain hormone-producing cells, particularly insulin-producing beta cells. Islet cells are harvested from the pancreas of a deceased donor and implanted into the portal vein of type 1 diabetics (Barker et al., 1990). While islets have been successfully injected into the liver portal vein allowing insulin-producing cells to restore blood glucose levels, islet infusion into the portal system has major drawbacks. The possibility of portal vein thrombosis or increased portal pressure after transplantation adds further complexity to the process (Baidal et al., 2003; Damyar et al., 2021). This is compounded by immunological reactions that reject and destroy the transplanted cells, as well as a scarcity of pancreas and islet donors, with early attempts to transplant pancreatic cells largely failing (de Klerk & Hebrok, 2021). Furthermore, most individuals with T1D require transplants from more than one donor to achieve clinically significant improvement. Because of these constraints, such therapies are limited to a small subset of patients suffering from severe hypoglycemia or unmanageable T1D (Loretelli et al., 2020). In order to protect the transplanted islets from the immune system, a lot of research has been invested in the development of immunosuppressive therapies. Despite advances in safer and more effective anti-inflammatory drugs, the need for long-term immunosuppression exposes transplant recipients to significant infections, cancer, and cellular damage (Ben Nasr et al., 2015; Shapiro et al., 2017).
Encapsulation procedures have been developed to improve islet transplantation by minimizing or delaying graft rejection. Encapsulation involves coating islets in a biocompatible semi-permeable membrane made of an inert material. This prevents the detection and attachment of immune cells and antibodies to the graft, which can delay rejection. Notably, the Diabetes Research Institute (DRI) in the United States is currently developing a bioengineered miniaturized pancreas where insulin-producing cells are encapsulated and implanted into the greater omentum (a highly vascularized region in the peritoneal cavity) (Pellicciaro et al., 2017). In 2016, the first patient in Europe was successfully treated with this approach, no longer requiring insulin therapy (Diabetes Research Institute Foundation, 2016). Significant obstacles to islet transplantation have highlighted the importance of finding alternatives to human tissue donors. The development of insulin-producing cells from stem cells, i.e. cells that have not yet differentiated into one of the numerous cell types in the human body, is a possible approach to curing T1D (Prochazkova et al., 2015). Vertex's VX-880 therapy, a first-of-its-kind form of stem cell therapy, aims to restore the body's ability to produce insulin by using functioning stem cell-derived beta cells (Garza & Hopcroft, 2021). The first transplant using VX-880 therapy successfully restored endogenous insulin production in a T1D patient in 2021, resulting in unprecedented insulin independence at day 270 post-treatment (Vieira, 2022). The ability to generate transplantable, insulin-producing cells removes the hurdle of relying on organ donors, making the VX-880's success a turning point in history; However, since these cells are generated outside of the patient's body, the patient also needs immunosuppression therapy, which is the major disadvantage of such state-of-the-art therapy.

Conclusions
Despite tremendous breakthroughs in disease treatment and management, diabetes poses a severe morbidity and mortality burden. This is especially true for people with T1D, whose quality of life is considerably decreased due to disease's challenging management. Although diabetes care has made tremendous strides since the discovery of insulin 100 years ago, there is still no cure for T1D. Several cutting-edge methods, including brand-new insulin formulas and ground-breaking devices, have been developed in an effort to cure diabetes. The advent of more precise insulin pumps and continuous glucose monitoring technology has not only improved glucose control, but has also enhanced patients' quality of life. The development of the first artificial pancreas capable of reducing the risk of hypo- and hyperglycemic crises was made possible by the integration of these devices with control algorithms. However, complete independence from exogenous insulin can only be attained through biological approaches that envision the replacement of functioning beta cells generated from stem cells: this will be a tremendous challenge, but also the greatest hope for individuals with T1D.
Bibliographical References
Albisser, A. M., Leibel, B. S., Ewart, T. G., Davidovac, Z., Botz, C. K., & Zingg, W. (1974). An Artificial Endocrine Pancreas. Diabetes, 23(5), 389–396. https://doi.org/10.2337/diab.23.5.389
Alcántara-Aragón, V. (2019). Improving patient self-care using diabetes technologies. Therapeutic Advances in Endocrinology and Metabolism, 10, 204201881882421. https://doi.org/10.1177/2042018818824215
American Diabetes Association. (2009). Diagnosis and Classification of Diabetes Mellitus. Diabetes Care, 32, S62–S67. https://doi.org/10.2337/dc09-S062
American Diabetes Association. (2017). Standards of Medical Care in Diabetes—2017 Abridged for Primary Care Providers. Clinical Diabetes, 35(1), 5–26. https://doi.org/10.2337/cd16-0067
Aroda, V. R., & Ratner, R. E. (2018). Metformin and Type 2 Diabetes Prevention. Diabetes Spectrum : A Publication of the American Diabetes Association, 31(4), 336–342. https://doi.org/10.2337/ds18-0020
Baeshen, N. A., Baeshen, M. N., Sheikh, A., Bora, R. S., Ahmed, M. M. M., Ramadan, H. A. I., Saini, K. S., & Redwan, E. M. (2014). Cell factories for insulin production. Microbial Cell Factories, 13(1), 141. https://doi.org/10.1186/s12934-014-0141-0
Baidal, D. A., Froud, T., Ferreira, J. V., Khan, A., Alejandro, R., & Ricordi, C. (2003). The Bag Method for Islet Cell Infusion. Cell Transplantation, 12(7), 809–813. https://doi.org/10.3727/000000003108747280
Barker, M., Starzl, T., & Tzakis, A. (1990). Pancreatic islet transplantation. The Lancet, 336(8726), 1323. https://doi.org/10.1016/0140-6736(90)93009-E
Bell, A. M. (2023). How insulin and glucagon regulate blood sugar. Medical News Today.https://www.medicalnewstoday.com/articles/316427#:~:text=Insulin%20reduces%20the%20body's%20blood,blood%20sugar%20levels%20to%20rise.
Ben Nasr, M., D’Addio, F., Usuelli, V., Tezza, S., Abdi, R., & Fiorina, P. (2015). The rise, fall, and resurgence of immunotherapy in type 1 diabetes. Pharmacological Research, 98, 31–38. https://doi.org/10.1016/j.phrs.2014.07.004
Biggers, A. (2022). Type 1 and Type 2 Diabetes: What’s the Difference? Healthline. https://www.healthline.com/health/difference-between-type-1-and-type-2-diabetes
Bluestone, J. A., Herold, K., & Eisenbarth, G. (2010). Genetics, pathogenesis and clinical interventions in type 1 diabetes. Nature, 464(7293), 1293–1300. https://doi.org/10.1038/nature08933
Brown, G. (2018). Abbott’s Freestyle Libre gains CE mark in Europe. Beyond Type 1. https://beyondtype1.org/abbotts-freestyle-libre-gains-ce-mark-in-europe/
Caccomo, S. (2018). FDA approves automated insulin delivery and monitoring system for use in younger pediatric patients. Food and Drug Administration (FD!). https://www.fda.gov/news-events/press-announcements/fda-approves-automated-insulin-delivery-and-monitoring-system-use-younger-pediatric-patients
Continuous Glucose Monitoring. (2017). National Institute of Diabetes and Digestive and Kidney Diseases. https://www.niddk.nih.gov/health-information/diabetes/overview/managing-diabetes/continuous-glucose-monitoring
Damyar, K., Farahmand, V., Whaley, D., Alexander, M., & Lakey, J. R. T. (2021). An overview of current advancements in pancreatic islet transplantation into the omentum. Islets, 13(5–6), 115–120. https://doi.org/10.1080/19382014.2021.1954459
Dansinger, M. (2022). Diabetes Insulin Pump: How It Works? WebMD. https://www.webmd.com/diabetes/insulin-pump
de Klerk, E., & Hebrok, M. (2021). Stem Cell-Based Clinical Trials for Diabetes Mellitus. Frontiers in Endocrinology, 12, 631463. https://doi.org/10.3389/fendo.2021.631463
Diabetes. (2023). NHS. https://www.nhs.uk/conditions/diabetes/
Diabetes Research Institute Foundation. (2016). First Type 1 Diabetes Patient in Europe is Free from Insulin Therapy after Undergoing Diabetes Research Institute’s BioHub Transplant Technique. PR Newswire. https://www.prnewswire.com/news-releases/first-type-1-diabetes-patient-in-europe-is-free-from-insulin-therapy-after-undergoing-diabetes-research-institutes-biohub-transplant-technique-300282581.html
Fernández, C. R. (2019). The Three Steps Needed to Fully Automate the Artificial Pancreas. Labiotech. https://doi.org/https://www.labiotech.eu/in-depth/artificial-pancreas-diabetes/
Fowler, M. J. (2008). Microvascular and Macrovascular Complications of Diabetes. Clinical Diabetes, 26(2), 77–82. https://doi.org/10.2337/diaclin.26.2.77
Garza, M., & Hopcroft, A. (2021). Hope Stems from Vertex’s New Therapy. DiaTribe. https://diatribe.org/hope-stems-vertex%E2%80%99s-new-therapy
Harris, T. (2023). What Is A Glucometer? Types, Benefits And Costs. Forbes Health. https://www.forbes.com/health/healthy-aging/glucometers-guide/
Hayes, C. (2020). How Do CGMs Work? [+ Tips for calibration]. Nutrisense. https://www.nutrisense.io/blog/how-cgms-work-and-accuracy
Illa, M. C., & Sańchez, F. S. (1975). Hyper and hypoglycemic coma. La Prensa Medica Mexicana, 40(1–2), 43–48. http://www.ncbi.nlm.nih.gov/pubmed/1144294
Jensen, T., & Deckert, T. (1992). Diabetic retinopathy, nephropathy and neuropathy. Generalized vascular damage in insulin-dependent diabetic patients. Hormone and Metabolic Research. Supplement Series, 26, 68–70. http://www.ncbi.nlm.nih.gov/pubmed/1490695
Kumar, A., Gangwar, R., Ahmad Zargar, A., Kumar, R., & Sharma, A. (2023). Prevalence of diabetes in India: A review of IDF Diabetes Atlas 10th edition. Current Diabetes Reviews, 19. https://doi.org/10.2174/1573399819666230413094200
Loretelli, C., Assi, E., Seelam, A. J., Ben Nasr, M., & Fiorina, P. (2020). Cell therapy for type 1 diabetes. Expert Opinion on Biological Therapy, 20(8), 887–897. https://doi.org/10.1080/14712598.2020.1748596
McCall, A. L. (2012). Insulin therapy and hypoglycemia. Endocrinology and Metabolism Clinics of North America, 41(1), 57–87. https://doi.org/10.1016/j.ecl.2012.03.001
Pellicciaro, M., Vella, I., Lanzoni, G., Tisone, G., & Ricordi, C. (2017). The greater omentum as a site for pancreatic islet transplantation. CellR4-- Repair, Replacement, Regeneration, & Reprogramming, 5(3). http://www.ncbi.nlm.nih.gov/pubmed/33834082
Porch, A. (2023). Continuous Glucose Monitoring (CGM) – What Is It? NUVI Health and Wellbeing. https://mynuvi.co/latest-updates/what-is-continuous-glucose-monitoring/
Prochazkova, M., Chavez, M. G., Prochazka, J., Felfy, H., Mushegyan, V., & Klein, O. D. (2015). Embryonic Versus Adult Stem Cells. In Stem Cell Biology and Tissue Engineering in Dental Sciences (pp. 249–262). Elsevier. https://doi.org/10.1016/B978-0-12-397157-9.00020-5
Reed, J., Bain, S., & Kanamarlapudi, V. (2021). A Review of Current Trends with Type 2 Diabetes Epidemiology, Aetiology, Pathogenesis, Treatments and Future Perspectives. Diabetes, Metabolic Syndrome and Obesity: Targets and Therapy, Volume 14, 3567–3602. https://doi.org/10.2147/DMSO.S319895
Satoh, T. (2014). Molecular Mechanisms for the Regulation of Insulin-Stimulated Glucose Uptake by Small Guanosine Triphosphatases in Skeletal Muscle and Adipocytes. International Journal of Molecular Sciences, 15(10), 18677–18692. https://doi.org/10.3390/ijms151018677
Shapiro, A. M. J., Pokrywczynska, M., & Ricordi, C. (2017). Clinical pancreatic islet transplantation. Nature Reviews Endocrinology, 13(5), 268–277. https://doi.org/10.1038/nrendo.2016.178
Todd, J. A. (2010). Etiology of Type 1 Diabetes. Immunity, 32(4), 457–467. https://doi.org/10.1016/j.immuni.2010.04.001
Vieira, G. (2022). Stem cell therapy for type 1 diabetes: Patient achieved insulin independence on day 270. Beyond Type 1. https://beyondtype1.org/diabetes-stem-cell-trial-vertex-270/
Voss, A. (2022). Bionic pancreas improves type 1 diabetes management compared to standard insulin delivery methods. National Institute of Diabetes and Digestive and Kidney Diseases (NIDDK). https://www.nih.gov/news-events/news-releases/bionic-pancreas-improves-type-1-diabetes-management-compared-standard-insulin-delivery-methods#:~:text=A%20device%20known%20as%20a,multicenter%20clinical%20trial%20has%20found.
Wardian, J. L. (2017). Diabetes Cannot Be Controlled, But It Can Be Managed. Clinical Diabetes, 35(5), 329–330. https://doi.org/10.2337/cd16-0061
Visual Sources