Xenobiotics: From Environmental Pollutants to Drug Metabolism
Whether it is ingested, passed through the skin, inhaled, or injected directly into the bloodstream, chemicals enter our bodies on a daily basis. It can be in the form of nutrition, pharmaceuticals, cosmetic ingredients, or environmental pollutants (Figure 1). Rachel Carson, an American biologist (Figure 2), summarised this in the following quotation "As crude a weapon as the caveman's club, the chemical barrage has been hurled against the fabric of life" (Carson, 1962, p. 297). The term xenobiotic refers to a chemical substance that is not naturally occurring and isn't expected to exist within an organism. It can also include substances that are present in higher-than-normal concentrations. From various literature sources, we have also learned that it is also possible for natural compounds to become xenobiotics when they are taken up by another organism, as is the case with fish taken up by sewage outfalls or animals producing chemical defenses to protect themselves from predators. Therefore, it is essential to understand how xenobiotics are handled at the cellular level in order to learn how to cope with them. And following such information, attempts have been made to modify microorganisms by introducing genes that encode enzymes that metabolize specific xenobiotics into harmless compounds. These modified organisms will then be used to help dispose of various pollutants that contaminate the planet (Murray et al., 2009).
Xenobiotic metabolism is an important concept to understand and fundamental to pharmacology, therapeutics, toxicology, cancer management, and drug addiction. All these areas involve the administration of, or exposure to, xenobiotics. Generally, pharmaceutical chemicals will have some beneficial properties at the concentrations exposed, although some can be toxic, in their parent form or metabolite. For chemical safety evaluation and drug development, determining the distribution and metabolic fate of a compound is essential, as is physiological-based pharmacokinetics (PBPK). PBPK modeling is a mathematical modeling technique for predicting the Absorption, Distribution, Metabolism, and Excretion (ADME) of synthetic or natural chemical substances in humans and other animal species (Shaik & Khan, 2019).

Xenobiotics in the Environment
A xenobiotic compound that is present in the environment as a pollutant is called an environmental xenobiotic. Medications or pharmaceutical drugs are substances used to alter, diagnose, prevent and treat disease, health conditions, body structure, or function. Some active pharmaceutical ingredients (API) can enter the environment through various routes either as the parent compound or the pharmacologically active metabolite. Most pharmaceuticals are designed to have a beneficial effect on the organism to which they are administered, but many of them often pass into the environment and have unwanted effects. While environmental researchers have mainly concentrated on well-known environmentally hazardous chemicals used in agriculture and industry, API were passing into the environment and have been largely ignored for many years. However, with increasing technology to help in the separation and identification of multiple compounds in a mixture, API and their effects have received increasing attention (Daughton & Ternes, 1999).
A wide range of pharmaceuticals has been found in the environment, including painkillers (analgesics and anti-inflammatory drugs), antibiotics (antibacterial), anticonvulsant drugs, beta-blockers, blood lipid regulators, contrast media for X-rays, cytostatic drugs (chemotherapy), oral contraceptives, and veterinary pharmaceuticals (Heberer, 2002).

Xenobiotic Metabolism
The body removes xenobiotics by xenobiotic metabolism. This occurs primarily in the liver, where xenobiotics are deactivated and excreted. Excretion routes are urine, feces, breath, and sweat. The process of metabolizing these substances starts with enzymes found in the liver, which first activate these compounds by a chemical reaction, which is then followed by a secondary reaction to form more soluble products, which are then finally excreted through bile or urine (Patterson et al., 2010). One example of a group of enzymes involved in xenobiotic metabolism is called, cytochrome P450. These enzymes play crucial roles when developing new drugs because they are responsible for the breakdown of medications within our body. The Sonoran Desert fly, Drosophila mettleri, has a unique cytochrome P450 system that enables it to exploit its xenobiotic resistance to nest over a wide range of both soils moistened by rotting cacti and on necrotic patches.
The metabolism of xenobiotics can be seen in two phases. As mentioned before an important reaction in phase 1 is hydroxylation, which is induced by cytochrome P450 enzymes (Figure 3). Hydroxylation is defined as a chemical process that introduces a hydroxyl group to form a hydroxylated product. This reaction may stop the intended action of the specific drug or xenobiotic, though this is not always the case (Esteves et al., 2021).
In phase 2, the hydroxylated product or other activated compounds produced from phase 1 are converted by a similar type of enzymes to more polar products (Figure 3). This is achieved through a reaction called conjugation. During this reaction, the hydroxylated compound reacts with various types of acids (i.e. glucuronic acids or amino acids). This process increases their water solubility or polarity which helps in the overall excretion of the compounds from the body. Extreme non-polar xenobiotics persist in adipose tissue almost indefinitely if they are not excreted from the body. Some xenobiotics become biologically active or more toxic as a result of phase 1 metabolic reactions, instead of being excreted. These xenobiotics are known as "prodrugs" or "procarcinogens" respectively.
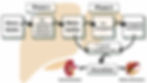
The term "detoxification" is sometimes used to describe many of the reactions involved in xenobiotic metabolism. Despite this, the term is not always applicable since, as mentioned above, sometimes xenobiotics' reactions actually increase their toxicity and biological activity (Esteves et al., 2021; Murray et al., 2009). Various factors such as age and sex affect the activities of xenobiotic-metabolizing enzymes. The activities may differ substantially among different species. A xenobiotic's potential toxicity or carcinogenicity, for example, cannot be expected to be the same when comparing data from one species to another.
The body's responses to different xenobiotics include pharmacologic, toxicologic, immunologic, and carcinogenic effects. These effects produced by drugs on the human body are referred to as the study of pharmacology; here it is important to understand that drugs work primarily through biochemical mechanisms. Pharmacogenetics on the other hand involves variations in drug response due to genetic makeup. In this area of science, genetic factors are studied in relation to drug response and toxicity. There are some xenobiotics that are toxic even at very low concentration levels (i.e. cyanide). On the other hand, there are very few xenobiotics that do not exhibit toxic effects. All drugs when administered in larger quantities will exhibit some sort of toxic response. In general, xenobiotics have three major toxic effects which can be divided into three categories as described in Figure 4. The figure shows how the metabolism of xenobiotics can result in cell injury, immunological damage, or cancer.

In Figure 4, the xenobiotic compound is converted to a reactive metabolite by a cytochrome P450 enzyme. The reactive metabolite (i.e. an epoxide compound) can then be transformed into a nontoxic metabolite by means of a different enzyme (i.e. Glutathione-S-transferase or by epoxide hydrolase) and excreted (Murray et al., 2009). If the reactive metabolite is not converted to a nontoxic substance, a different pathway (as seen in Figure 4) occurs. There are three different processes that can result. The first is cell injury also known as cytotoxicity, which can be severe enough to result in cell death. There are many mechanisms by which xenobiotics injure cells. The one considered here is where the xenobiotic binds to macromolecules within the cell in a covalent manner, which means they bind irreversibly, and the effects of this binding cannot be reversed. These macromolecular targets include deoxyribonucleic acid (DNA), ribonucleic acid (RNA), and proteins. If the macromolecule to which the xenobiotic binds is essential for short-term cell survival, i.e., a protein or enzyme involved in some critical cellular function, then severe effects could become evident quite rapidly.
The second is when the active form of a xenobiotic binds to a protein, altering its antigenicity. Antigenicity is when a chemical compound (either an antigen or hapten) binds specifically to a group of specialized, systemic cells that eliminate pathogens (an organism that causes disease to its host) or prevent their growth. The hapten or xenobiotic then reacts specifically with the antibodies generated against it to produce an immune or allergic response. Thus, although the hapten cannot elicit an antibody response on its own, it can bind with antibodies and elicit a response. Many drugs that cause allergic reactions, such as penicillin (Figure 5), act as haptens. When injected or ingested, penicillin reacts chemically with proteins in the body to form a carrier complex that elicits an allergic response, this can then lead to the life-threatening syndrome called anaphylaxis.
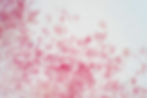
Finally, activated species of chemical carcinogens (a substance, organism, or agent capable of causing cancer) are thought to play a very important role in chemical carcinogenesis. Indirect carcinogens require activation by monooxygenases (a group of specific enzymes) within the endoplasmic reticulum to become cancerous (they are therefore called indirect carcinogens). Endoplasmic reticulum monooxygenases as well as other xenobiotic-metabolizing enzymes help to determine whether a compound becomes carcinogenic or "detoxified". Direct carcinogens (i.e., various alkylating agents) can directly interact with DNA without undergoing intracellular chemical activation. On the other hand, certain enzymes such as epoxide hydrolase exhibit a protective effect against certain carcinogens, because it has the ability to break down these carcinogens. Epoxides are seen as carcinogens, they are formed when certain cytochrome P450 enzymes react with xenobiotics that are pro-carcinogenic. Epoxides are usually highly reactive and mutagenic or carcinogenic or both. The enzyme, epoxide hydrolase, like cytochrome P450, converts these compounds (epoxides) into less reactive compounds (i.e. dihydrodiols) that are easily excreted in the bile and urine (Fretland & Omiecinski, 2000). The reaction catalyzed by the enzyme epoxide hydrolase is presented in Figure 6.
The development of new and safer drugs drives the development of pharmacogenetics, and understanding how genetic variability influences drug treatment outcomes. Advances in sequencing the human genome have also led to the development of a new field of study called pharmacogenomics. It has been defined as the process of identifying and developing drug targets and active pharmaceutical drugs using genomic information and technologies. It builds on pharmacogenetics, which covers a wider sphere of activity. By integrating genomics, proteomics, bioinformatics, biochemistry, and toxicology information, new and safer drugs can and will be developed in the future. New drug developments focus on improving treatment and providing safer, more personalized drugs, taking into account environmental and genetic factors. Approximately 100,000 Americans die each year from adverse drug reactions (Leaman et al., 2010). New information from studies in these and other areas will hopefully lead to successful therapies and eventually a new era of personalized medicine. However, much work remains to be done before this is achievable.

Conclusion
To conclude, the metabolism of xenobiotics is an intricate and fascinating process that determines how foreign substances are absorbed and metabolized within living organisms. It is imperative that metabolic pathways be intricate and efficient in order to effectively manage the diverse range of xenobiotics, which include both environmental pollutants and pharmaceuticals.
Xenobiotics undergo biotransformation through different stages of metabolism, which can result in detoxification, activation, or the formation of metabolites with altered properties. Xenobiotics undergo these transformations not only to influence their biological activity and toxicity but also to determine their distribution and elimination. Drug efficacy, drug-drug interactions, and drug-induced toxicity have been influenced by the study of xenobiotic metabolism. Furthermore, it has contributed significantly to our understanding of environmental pollution, as xenobiotic biodegradation pathways affect their persistence and environmental impact on different ecosystems. It is evident that individual variations in xenobiotics metabolism can influence an individual's response to drugs and environmental exposures.
Identifying novel enzymes, pathways, and mechanisms involved in xenobiotic metabolism remains a priority as we advance our metabolic understanding. In addition, cutting-edge technologies, such as metabolomics and computational modeling will help predict metabolic outcomes and optimize drug development. Ultimately, understanding the metabolism of xenobiotics can contribute to the improvement of drug safety, environmental protection, and public health. By unraveling the complexities of biotransformation pathways and utilizing this knowledge for risk assessment and drug design, everyone and the planet will be able to live a healthier and safer life.
Bibliographical References
Carson, Rachel. 1962. Silent Spring. Boston: Houghton.
Daughton, C. G., & Ternes, T. A. (1999). Pharmaceuticals and personal care products in the environment: agents of subtle change? Environmental health perspectives, 107, 907–938. DOI: http://dx.doi.org/10.1289/ehp.99107s6907
Esteves, F., Rueff, J., & Kranendonk, M. (2021). The central role of cytochrome P450 in xenobiotic metabolism - A brief review on a fascinating enzyme family. Journal of Xenobiotics, 11(3), 94–114. Retrieved July 28, 2023, from https://www.mdpi.com/2039-4713/11/3/7.
Fretland, A. J., & Omiecinski, C. J. (2000). Epoxide hydrolases: biochemistry and molecular biology. Chemico-biological interactions, 129(1–2), 41–59.
Heberer, T. (2002). Occurrence, fate, and removal of pharmaceutical residues in the aquatic environment: a review of recent research data. Toxicology letters, 131(1–2), 5–17.
Leaman, R., Wojtulewicz, L., Sullivan, R., Skariah, A., Yang, J., & Gonzalez, G. (2010). Towards internet-age pharmacovigilance: extracting adverse drug reactions from user posts in health-related social networks. Proceedings of the 2010 workshop on biomedical natural language processing, 117–125.
Murray, K., Rodwell, V., Bender, D., Botham, K. M., Weil, P. A., & Kennelly, P. J. (2009). Harper's illustrated biochemistry. 28. Citeseer, New York, United States, 609 –615.
Patterson, A. D., Gonzalez, F. J., & Idle, J. R. (2010). Xenobiotic metabolism: a view through the metabolometer. Chemical research in toxicology, 23(5), 851–860.
Shaik, A. N., & Khan, A. A. (2019). Physiologically based pharmacokinetic (PBPK) modeling and simulation in drug discovery and development. ADMET and DMPK, 7(1), 1–3. DOI: https://doi.org/10.5599/admet.667
Visual Sources