Mechanisms of Developmental Biology Series: How It All Begins
- Gülce Tekin
- Oct 26, 2023
- 17 min read
Updated: Sep 11, 2024
Foreword
The progression from a single-cell entity to a multicellular organism entails a series of intricate stages. This intricate journey involves the orchestrated activities of stem cells and the process of differentiation, which collectively constitute the life cycle of complex, multicellular organisms. The domain of developmental biology transcends human boundaries, encompassing studies of animals and plants alike, underscoring its extensive purview. The pursuit of unraveling the intricate mechanisms governing organismal development not only illuminates the origins of life but also provides invaluable insights into the molecular and cellular underpinnings of development across diverse species. The curriculum of this developmental biology series serves as an illuminating beacon, shedding light on the myriad manifestations of life on our planet. To gain a more profound comprehension of developmental processes, this series will traverse multiple interdisciplinary avenues, including embryology, medical science, and evolutionary biology. Through this holistic approach, we endeavor to uncover the underlying principles governing the diverse tapestry of life forms on Earth.
This series will be divided into the following sections:
1. Mechanisms of Developmental Biology Series: Essence of All
2. Mechanisms of Developmental Biology Series: How It All Begins
3. Mechanisms of Developmental Biology Series: Journey to Life
4. Mechanisms of Developmental Biology Series: Offshoot of a Plant
5. Mechanisms of Developmental Biology Series: Epagoge in Development: From Embryology to Evolution
6. Mechanisms of Developmental Biology Series: Cutting-edge Techniques in Developmental Biology
Mechanisms of Developmental Biology Series: How It All Begins
The journey from a single-celled zygote to a fully formed human being is a testament to the inspiring process of embryonic development. It all commences with the miraculous moment of fertilization, where a sperm cell embarks on an incredible journey to unite with an oocyte, marking the inception of a new life (Oliver & Basit, 2023). This journey through embryogenesis takes us beyond fertilization, unveiling a sequence of extraordinary processes, including activation of the egg, cleavage, gastrulation, and organogenesis, each contributing to the creation of a unique individual. These developmental milestones showcase the marvels of biology and the complexity of life's origins.
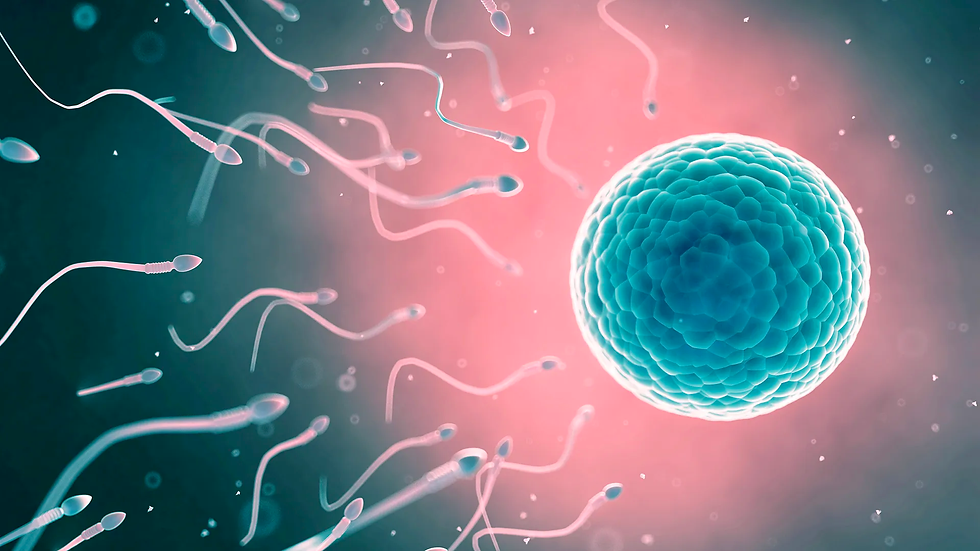
Fertilization
The process of development from the embryo to a single-celled zygote begins with fertilization, the intricate journey that a sperm cell undertakes to fuse with the oocyte, the female egg cell. At this moment, the pregnancy begins (Oliver& Basit, 2023). Within the zygote, the genetic information received from the parent cells is a complete set. In primitive organisms, such as microorganisms and protozoans, fertilization involves the exchange of genetic material between two cells. In advanced animals and humans, it is typically followed by the penetration of the egg by a single spermatozoon (Khan & Ackerman, 2023). With the initiation of fertilization, the egg becomes activated by the induction of a rise in intracellular calcium concentrations. This increase in calcium results in the transversion of a signaling wave through the egg, allowing it to undergo cell division by leaving meiotic arrest and metabolic dormancy (Marlon, 2010). After activation, the egg continues with Meiosis II by releasing the second polar body (non-functional cells with a reduced amount of genetic material, the main function is to ensure that the chromosome number of the fertilized egg is correct) (Schmerler & Wessel, 2011).
The increase in intracellular calcium levels also induces reorganization of the egg surface in order to prevent polyspermy, the entry of more than one sperm cell into the egg via a phenomenon called the cortical reaction. Another rearrangement includes changes in the cytoplasm before the first cleavage happens (Marlon, 2010). The activation of the egg does not necessarily require fertilization by a sperm cell. Parthenogenesis is a mode of asexual reproduction in which the egg cell contains all the genetic material necessary for the development of the offspring. Physical factors, such as temperature, pressure, or even mechanical stimulation, can serve as triggers for egg activation in parthenogenesis. For example, in some species such as Parus major (the great tit), changes in temperature may signal the egg to start developing (Bleu et al., 2019). Production of natural chemicals by other organisms might also trigger the activation process.
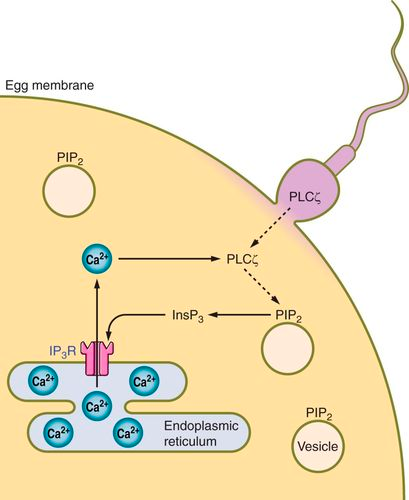
The acrosome is a specialized cap-like structure at the front tip of a sperm cell, containing enzymes and other substances necessary for fertilization. The acrosome reaction is a crucial step in the process of fertilization, enabling the spermatozoon to penetrate and fuse with the egg. This reaction makes sure that the entry of the sperm cell is initiated via penetration into the layers surrounding the egg. When the sperm cell comes into contact with the zona pellucida, which is an outer layer surrounding the egg, specific receptors on the sperm's surface interact with molecules in the zona pellucida (Alberts et al., 2002). Within the acrosome, a structure called the acrosomal vesicle bursts, leading to the formation of an opening at the tip of the sperm. This opening allows the release of the acrosomal enzymes into the zona pellucida, weakening the protective barrier of the egg and digesting the molecules within it. With the help of the cortical granules, the vitelline membrane rises from the egg surface to form the fertilization membrane, which is a well-organized molecular structure that acts as a physical and biochemical barrier to prevent other sperm cells from entering the egg once the first sperm has successfully fertilized it (Eddy & Shapiro, 1979). This helps ensure that the resulting zygote has the correct genetic information. The plasma membrane of the fertilized egg undergoes changes, and a change in electric charge occurs across the plasma membrane. The sperm cell then follows the path created, reaching the egg cell membrane to initiate fusion via the merging of the cell membranes of the two cells (Alberts et al., 2002).
During the process of fertilization, the sperm nucleus, which contains the genetic material from the male, enters the egg cytoplasm where the egg nucleus is also present. The dormant sperm nucleus undergoes significant morphological changes such as the swelling and the expanding of the nucleus as well as the disintegration of the membranous envelope (Georgadaki et al., 2016). The genetic material within the sperm nucleus becomes organized and aligned in a manner similar to the female pronucleus, and centrioles begin to appear, allowing the two nuclei to fuse together, resulting in the formation of a zygote nucleus that has the genetic material from both of the parents. The transition from fertilization to cleavage is primarily triggered by the activation of mitosis-promoting factor (MPF) (Kishimoto, 2015).
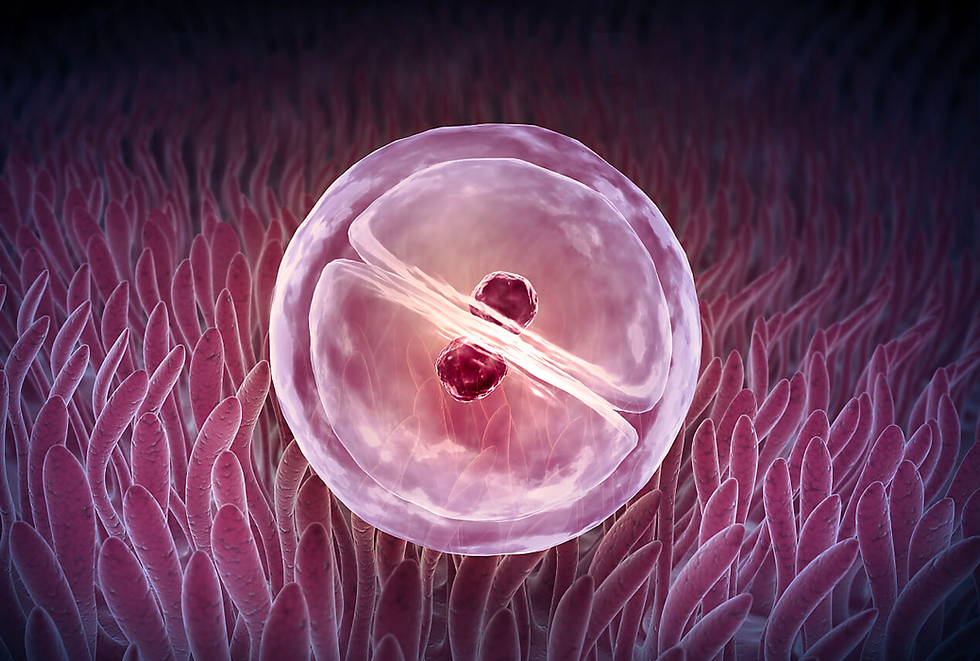
Cleavage
Cleavage is the early series of rapid and successive mitotic divisions that follow fertilization in the development of a multicellular organism. The result of these divisions is the parting apart of the zygote into smaller cells called “blastomeres”. In the majority of species except mammals, these divisions are arranged entirely by maternal resources such as maternal proteins and messenger RNAs (mRNAs) preloaded in the oocyte during oogenesis. These control the rate of cell division and the arrangement of blastomeres in relation to each other, while the zygotic genome remains largely dormant during the early stages of cleavage. Some mRNAs are actively transcribed until later in the cleavage process, and experimental transcription inhibition doesn't impede the proper division of the embryo. During cleavage, despite the division of the egg into progressively smaller cells, there is no substantial increase in cytoplasmic volume compared to nuclear volume, achieved by eliminating the growth periods, G1 and G2, of the cell cycle (Setti et al., 2018). Concurrently, the cleavage of nuclei occurs at a remarkably rapid pace, surpassing even that observed in tumor cells.
Cleavage is fundamentally a result of two coordinated processes. Firstly, karyokinesis, which refers to the mitotic division of the nucleus, is powered by the mitotic spindle—a structure composed of microtubules made of tubulin, the same protein found in the sperm flagellum. The second process is cytokinesis, which involves the division of the cell and is facilitated by a contractile ring of microfilaments composed of actin. Actin is the same protein that extends the microvilli in the egg and the acrosomal reaction in the sperm. The interplay and coordination between these two systems can be observed in cleavage, with the mitotic spindle and contractile ring positioned perpendicular to each other. The contractile ring creates a cleavage furrow, ultimately bisecting the plane of mitosis and generating two genetically equivalent blastomeres.
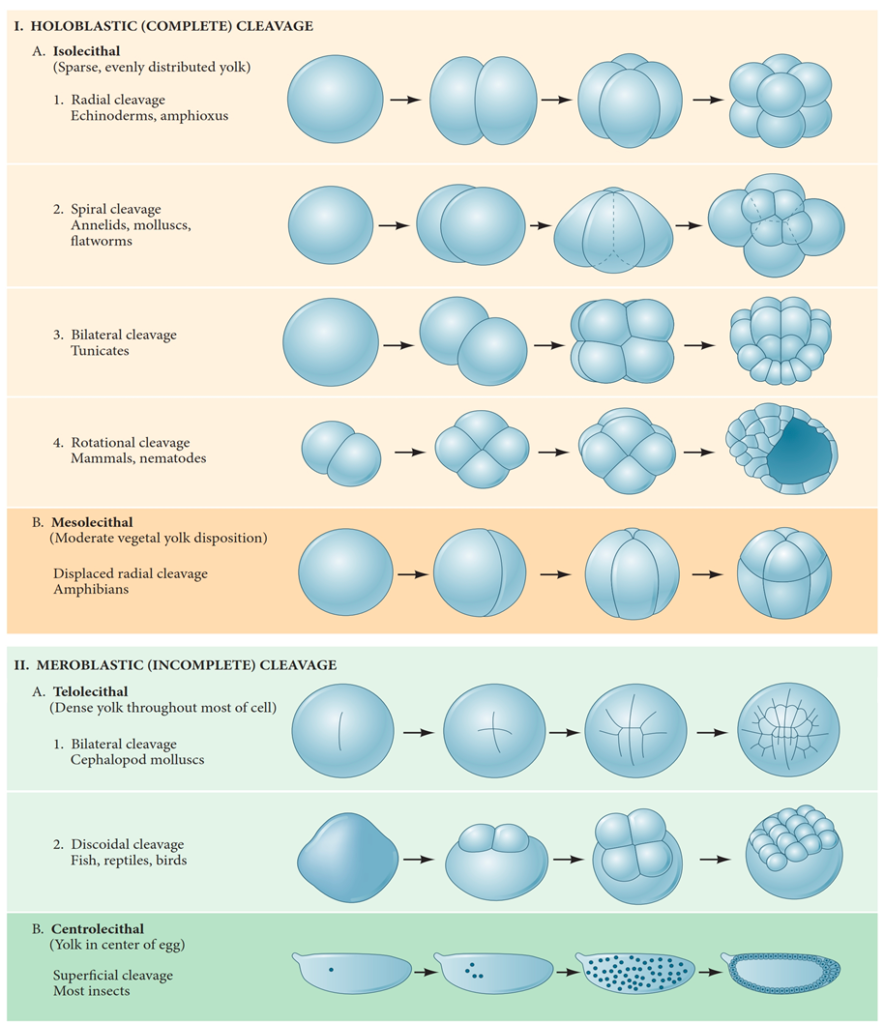
Another significant factor impacting cleavage is the distribution of yolk within the egg. The distribution of yolk in the egg significantly influences the pattern of cleavage, with various species adapting their cleavage patterns according to their unique environmental and nutritional needs, influencing the relative sizes of the blastomeres. In eggs with a relatively yolk-free pole (the vegetal pole), cell divisions occur more rapidly than at the yolk-rich pole (the animal pole) (Gilbert, 2000). Besides the yolk concentration playing a vital role, genetic factors also enhance specific patterns of cell division on top of these yolk-related constraints. Even in eggs with minimal yolk content, four major types of cleavage patterns can be observed: radial holoblastic, spiral holoblastic, bilateral holoblastic, and rotational holoblastic cleavage. These patterns, influenced by both genetic inheritance and the presence of yolk, contribute to the diversity of cleavage observed in different species (Hasley et al., 2017).
Gastrulation
Gastrulation stands as an essential morphogenetic program in animal embryogenesis, serving three fundamental objectives that significantly influence an organism's development in the early stages. The first task of gastrulation is associated with establishing the primary body axis, including the anteroposterior (head to tail) and dorsoventral (back to belly) axes (see Figure 6). The initiation of the establishment of these axes happens during gastrulation. Through a series of complex morphogenetic movements, such as epibolic movements (which lead to the spreading and thinning of germ layers, a critical process in shaping the embryo), cells undergo specific transformations that contribute to the determination of the main body axis. Another example would be the convergence movements that further narrow germ layers dorsoventrally, while concurrent extension movements cause anteroposterior elongation. These movements, along with other cell behaviors, result in the generation of the primary body axes (Anlas & Trivedi, 2021).

Simultaneously, gastrulation orchestrates the intricate patterning of the germ layers while guiding the internalization of mesodermal and endodermal precursors. During gastrulation, the mesodermal and endodermal cells, which are precursors of specific tissues and organs, undergo internalization, meaning that they move from their original positions to new locations within the embryo (Solnica-Krezel & Sepich, 2012). This process is strictly coordinated through the specific movements of two distinct cell types: epithelial and mesenchymal cells, which can be characterized by their unique morphological and functional attributes. Epithelial cells form cohesive layers, firmly adhering to one another through intercellular adhesion complexes, such as tight junctions, adherens junctions, desmosomes, and gap junctions along their lateral membrane. These cells exhibit apico-basal polarity, featuring a characteristic basal localization of a basement membrane that separates the epithelium from adjacent tissues. In contrast, mesenchymal cells lack polarity and do not form intercellular junctions, allowing them to exist as independent entities, capable of individual movement within the extracellular matrix (Thiery & Sleeman, 2006).
The third fundamental objective of gastrulation in animal embryogenesis is the internalization of mesodermal and endodermal precursors. This process involves the movement of cells from the surface of the embryo to the interior, ultimately leading to the formation of multiple germ layers and the organization of body structures. The process of internalization ensures that the cells are correctly positioned within the developing embryo, providing a route for their differentiation into the various tissues and organs they will become, such as muscles, bones, internal organs, connective tissues, etc. Notably, the process of internalization plays a pivotal role in shaping the course of gastrulation.
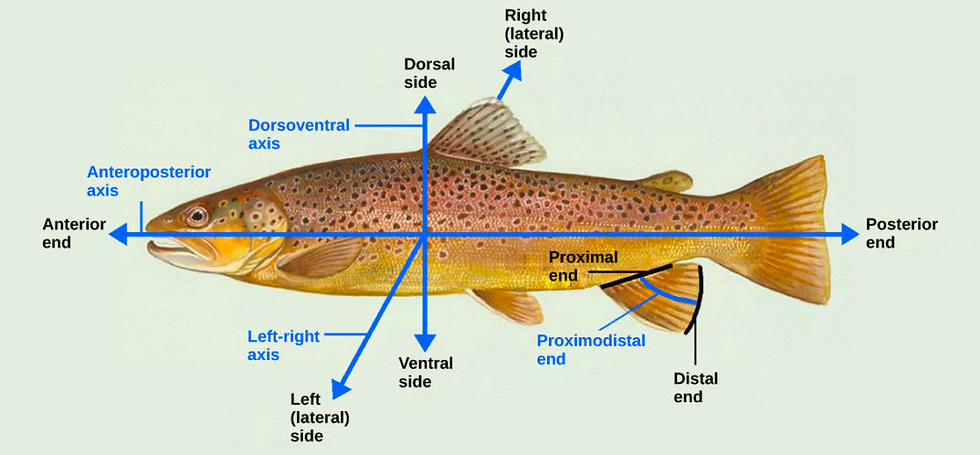
In most invertebrates and amniotes, mesodermal internalization occurs through the bending of the blastoderm inwards (invagination), exemplified by the processes observed in sea urchins and Drosophila melanogaster. Alternatively, these internalization movements can take the form of rolling through a slit-shaped opening (involution), as observed in Xenopus. Conversely, in amniotes such as reptiles, birds, and mammals, mesoderm precursors adopt a mesenchymal phenotype and ingress into the embryo as individual cells, as seen in mouse and chick embryos (Serrano & Weijer, 2023). There are two main modes of internalization: involution or invagination, in which mesodermal precursors move as a coherent epithelial layer, and ingression, which allows the epiblast (the outermost cell layer of the embryo) to remain flat. These different modes play a critical role in determining the overall shape and morphology of the developing embryo.
During gastrulation, the internalization of mesodermal and endodermal precursors necessitates an epithelial-to-mesenchymal transition (EMT), in which cells transition from a typical epithelial state with characteristics like apicobasal polarity and tight junctions into a mesenchymal state characterized by greater motility and more irregular morphology. This transition can be seen as a gradient, with fully epithelial cells having limited motility, and fully mesenchymal cells displaying high motility and individual migration. The changes between the epithelial and mesenchymal cell phenotypes can be reversed (Thiery & Sleeman, 2006). The extent to which mesodermal precursors undergo EMT is a major determinant of gastrulation morphology, influencing whether cells remain coherent within an epithelial layer, glide over each other during involution, or ingress individually. This process of EMT is essential for germ layer formation and cell migration in the early vertebrate embryo. Upon undergoing EMT, the cells acquire migratory and invasive properties that allow them to migrate through the extracellular matrix.
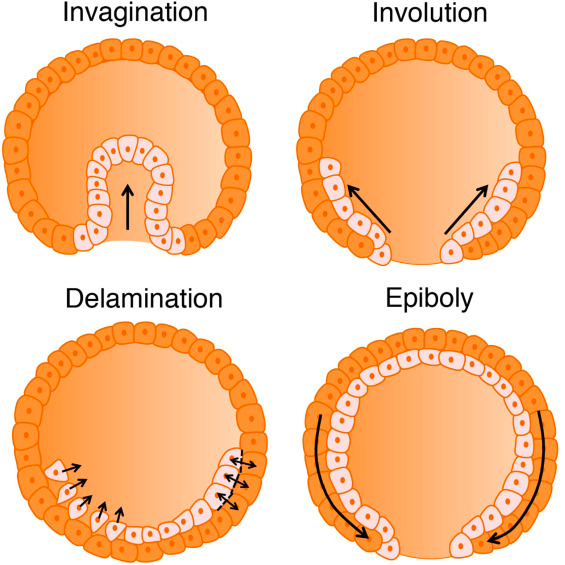
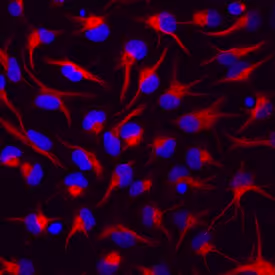
Organogenesis
Organogenesis marks a significant phase in the complex journey of embryonic development, where an organism's essential organs take shape and intricate processes unfold to lay the foundation for life. In the span of weeks six to eight, a symphony of organ development is conducted, each system with its unique and complex set of processes.
Cardiovascular System
The first signs of developmental orchestration are seen in the cardiovascular system: by the end of week four, a feat of nature occurs as the heart remarkably forms its four chambers. During week six, the cardiac outflow separation takes place, and the heart and lungs descend into the thorax. This critical event is not only a separation but also a transformation, involving the truncus arteriosus (aorticopulmonary trunk). Through a mesmerizing process of spiraling, the truncus arteriosus gives rise to the ascending aorta and pulmonary artery. The aorta and pulmonary artery appear to wind around each other superior to the heart, a testament to the profoundness of embryonic development (Donovan & Cascella, 2022).
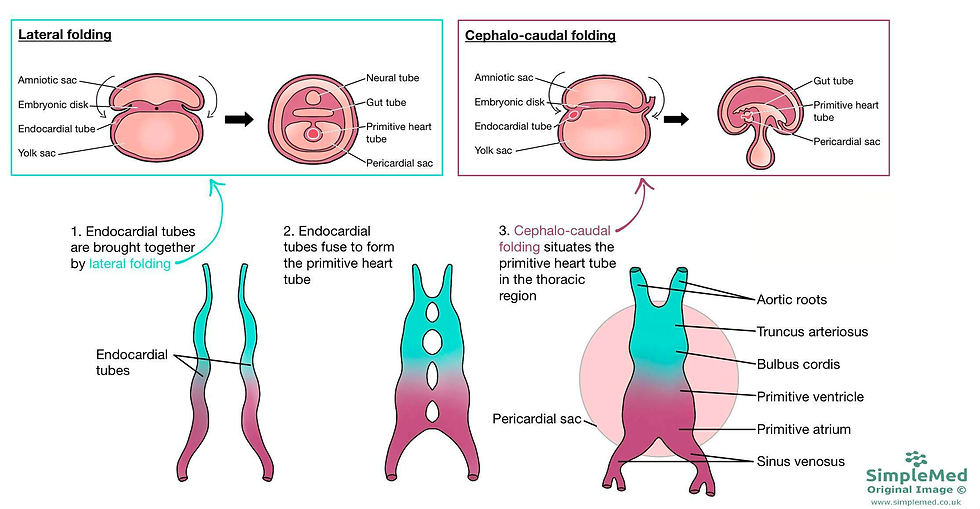
Lung Development
Lung development occurs from the embryonic period through the fetal period and continues up to birth. In particular, lung growth begins in early embryonic development when right and left lung buds are formed from an initial out-pouching, the respiratory diverticulum. The buds enlarge and branch to form the respiratory tree. After further maturation, the visceral and parietal pleura are formed during weeks five through seven. The visceral pleura covers the developing bronchial tree, and the parietal pleura covers the internal chest wall. Pleuroperitoneal membranes form and fuse with the diaphragm, which separates the pleural and peritoneal body cavities. Closure of the pleuroperitoneal canal by these membranes takes place by approximately week seven (Donovan& Cascella, 2022).
Gastrointestinal Development
During the early weeks of embryonic development, a remarkable process unfolds as the primitive gut tube takes shape. This pivotal development occurs as the embryo undergoes craniocaudal (head-to-tail) and lateral (side-to-side) folding, ultimately incorporating the yolk sac into the emerging gut structure. The primitive gut tube, divided into three distinct sections, is a fundamental foundation for the future gastrointestinal system. These three segments are the foregut, midgut, and hindgut, each with its unique role and contributions to organ formation.
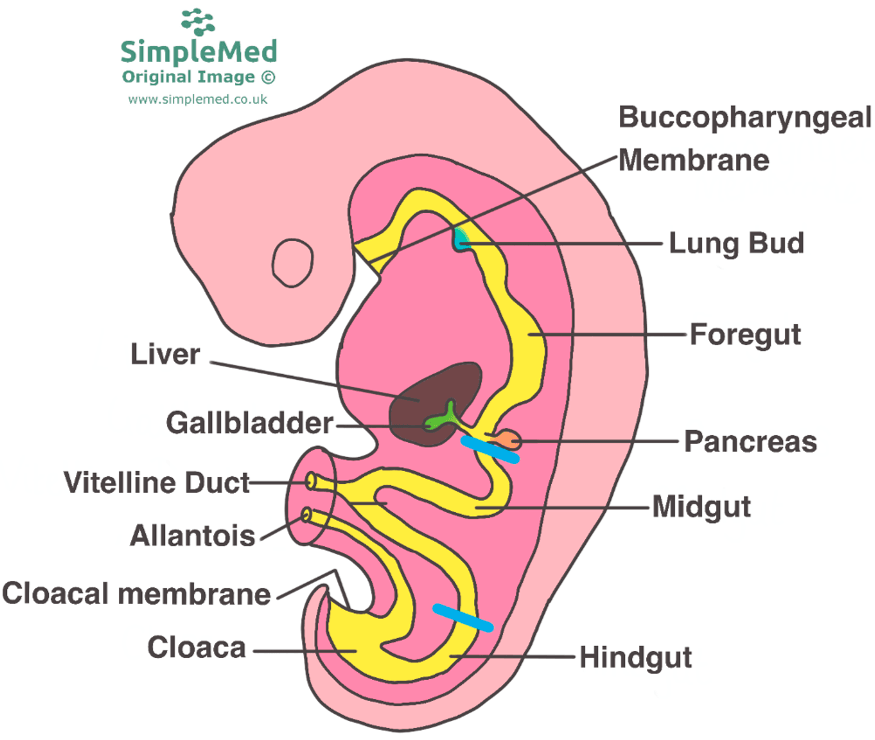
The foregut is responsible for giving rise to several vital organs and structures that play essential roles in digestion and metabolism. Within the foregut, precursors for the esophagus are observed, the tubular structure connecting the mouth to the stomach. It also serves as the foundation for the development of the stomach, a key organ for the initial stages of food digestion (Rao & Wang, 2010). Furthermore, the foregut is the origin of the liver, a central hub for metabolic functions, and the gallbladder, which stores bile produced by the liver. The bile ducts, responsible for transporting bile, and the pancreas, an organ pivotal in both endocrine and exocrine functions, also arise from this section. Finally, the proximal duodenum, the initial part of the small intestine that continues digestion, stems from the foregut.
The midgut is another significant portion of the primitive gut tube, with its own distinct contributions to the gastrointestinal system (Bhatia et al., 2023). Within the midgut, the development of crucial segments of the small intestine, including the distal duodenum, jejunum, and ileum are observed. These regions are primary sites for nutrient absorption, playing a vital role in nourishing the body. Additionally, the midgut forms the cecum, the initial part of the large intestine, and its appendix, a structure that still holds some mysteries regarding its exact function. As development progresses, the midgut also contributes to the formation of the ascending colon, a section of the large intestine, as well as the proximal two-thirds of the transverse colon.
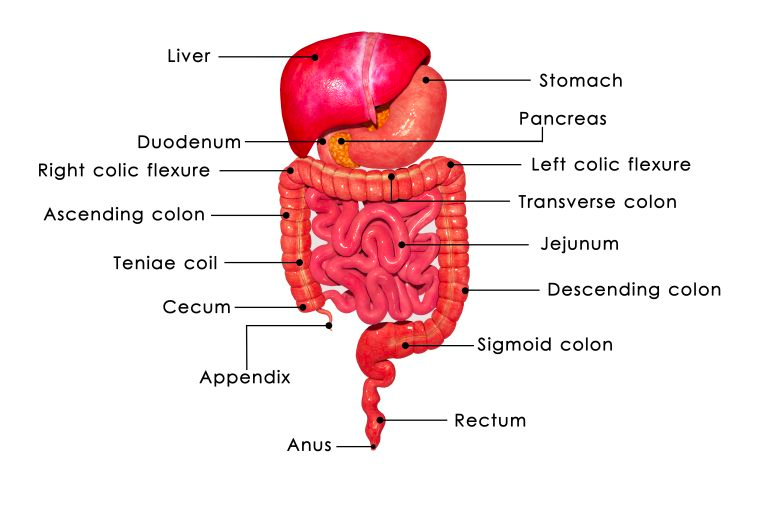
The hindgut represents the last segment of the primitive gut tube, and it also plays a significant role in the overall development of the gastrointestinal system. It gives rise to the distal one-third of the transverse colon, a region that will later be involved in processing waste and reabsorbing water. As development continues, the hindgut leads to the formation of the descending colon, which is integral in the movement and storage of fecal material, and the sigmoid colon, a curved part of the large intestine. Lastly, the hindgut contributes to the creation of the upper anal canal, marking the end of the gastrointestinal tract (Bhatia et al., 2023).
This process of segmenting the primitive gut tube into foregut, midgut, and hindgut is a testament to the precision and complexity of embryonic development. Each of these segments ultimately gives rise to specific organs and structures that collectively form the elaborate and highly functional human gastrointestinal system. This system is crucial for digestion, absorption of nutrients, and waste elimination, supporting life from the very beginning of humans’ existence.
Central Nervous System
The process of the central nervous system (CNS) development happens from week five through week eight, setting the stage for the complex structures of the brain and spinal cord. As the neural tube, the early foundation of the CNS, closes around Week 4, it marks the beginning of a sequence of events that will shape the human brain. During this critical period of embryonic development, the CNS undergoes a transformative process where specialized regions begin to take form. At the heart of this process is the development of vesicles, which are embryologic precursors to various structures within the brain that will eventually evolve into distinct parts of the CNS (Elshazzly et al., 2023).
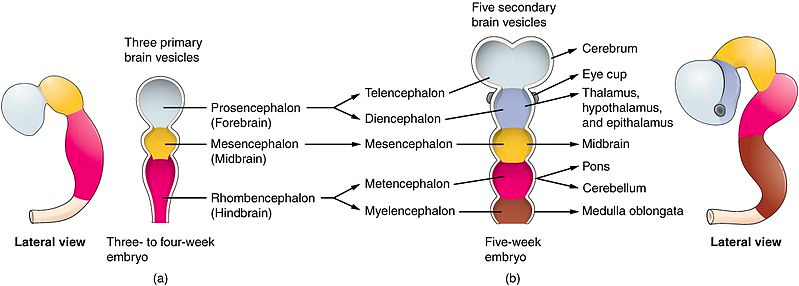
The prosencephalon, often referred to as the forebrain, is one of the primary subdivisions that emerge from these vesicles. It serves as the foundation for the development of two significant components: the diencephalon and the telencephalon. The diencephalon, an essential part of the forebrain, is the origin of structures such as the thalami, which play a central role in sensory processing, as well as the hypothalamus, responsible for regulating numerous vital functions. Additionally, the optic cups, crucial for vision, and the neurohypophysis, an integral component of the pituitary gland, are also derived from the diencephalon (National Research Council (US) Subcommittee on Reproductive and Neurodevelopmental Toxicology, 1989). Simultaneously, the telencephalon begins to grow, eventually enveloping the diencephalon, midbrain, and hindbrain.
The mesencephalon is another important structure that begins to form during this period. It contributes to various essential components within the brain. Among its creations, there is the aqueduct of Sylvius, a fluid-filled canal that plays a crucial role in cerebrospinal fluid circulation. Furthermore, the mesencephalon is responsible for the development of the superior and inferior colliculi, which are associated with sensory processing, particularly in the context of vision and hearing. Additionally, the tegmentum, an integral part of the brain's ventral region, is also formed from the mesencephalon.

The final structure that gets formed during this period of CNS development is the hindbrain, often referred to as the rhombencephalon. It gives rise to the fourth ventricle, a fluid-filled cavity within the brain. This structure serves various functions, including the production and circulation of cerebrospinal fluid. Moreover, the rhombencephalon paves the way for the development of the metencephalon (National Research Council (US) Subcommittee on Reproductive and Neurodevelopmental Toxicology, 1989). This crucial structure eventually matures into the pons, which is involved in several essential functions, and the cerebellum, responsible for coordinating voluntary movements and maintaining balance.
This meticulous process of vesicle formation and its subsequent development into distinct brain structures highlights the complexity of embryonic CNS development. As these early structures take shape, they set the stage for the functions of the human brain, playing a central role in everything from sensory processing to maintaining balance and orchestrating voluntary movements.
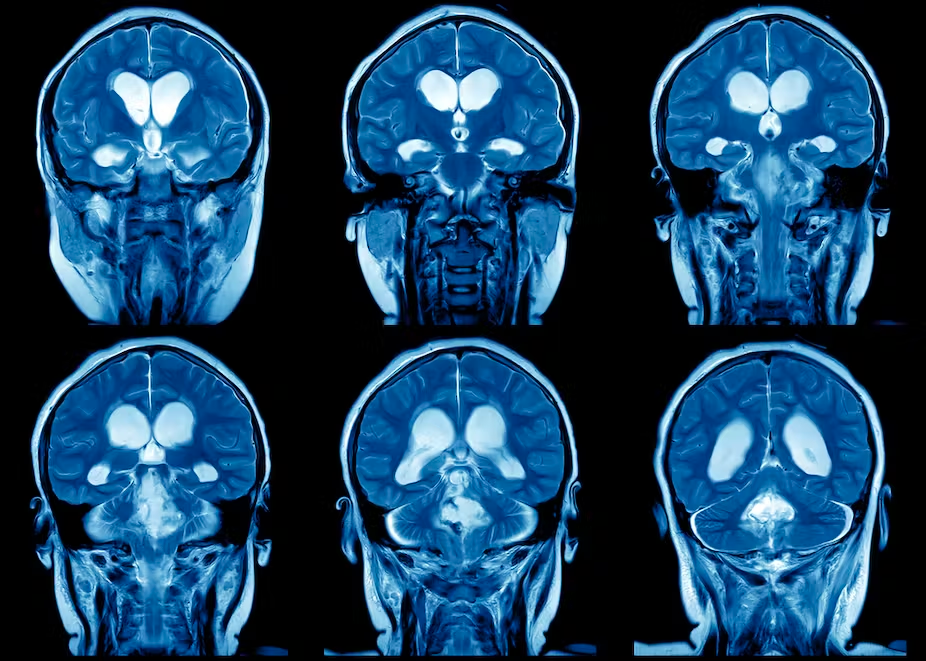
Other Organs
Many other organs such as the pituitary gland, thymus, and adrenal gland during Weeks 6-8. At week seven, the embryo gets into a characteristic C-shape and the ocular retina also begins to develop. The upper and lower limbs continue to grow along with the emergence of facial structures such as the nostrils, eyelids, outer ears, lip, and palate, and at week seven, the head and face contours (Donovan & Cascella, 2022).
Conclusion
Embryonic development, from fertilization through cleavage and gastrulation to organogenesis, is a marvel of nature. It is a journey characterized by intricate molecular choreography, complex cellular movements, and precise genetic instructions. The orchestration of this journey involves a symphony of events that contribute to the formation of the complex human body. Throughout the early stages of development, specific landmarks, such as the establishment of the primary body axes during gastrulation or the development of vital organ systems, define the trajectory of an individual's life. Understanding these milestones not only provides insights into the biology of life but also underscores the beauty and complexity of the natural world. The journey from a single fertilized egg to a fully developed human being is a testament to the wonders of biology and the miracle of life itself.
Bibliographical References
Alberts B, Johnson A, Lewis J, et al. (2002). Fertilization.Molecular Biology of the Cell. 4th edition. New York: Garland Science; Available from: https://www.ncbi.nlm.nih.gov/books/NBK26843/
Anlas, K., & Trivedi, V. (2021). Studying evolution of the primary body axis in vivo and in vitro. eLife, 10, e69066. https://doi.org/10.7554/eLife.69066
Bleu J., Agostini S., Angelier F., Biard C. (2019). Experimental increase in temperature affects eggshell thickness, and not egg mass, eggshell spottiness or egg composition in the great tit (Parus major), General and Comparative Endocrinology,Volume 275,Pages 73-81,ISSN 0016-6480,
https://doi.org/10.1016/j.ygcen.2019.02.004.
Bhatia A, Shatanof RA, Bordoni B. Embryology, Gastrointestinal. [Updated 2023 May 1]. In: StatPearls [Internet]. Treasure Island (FL): StatPearls Publishing; 2023 Jan-. Available from: https://www.ncbi.nlm.nih.gov/books/NBK537172/
Donovan MF, Cascella M. Embryology, Weeks 6-8. [Updated 2022 Oct 10]. In: StatPearls [Internet]. Treasure Island (FL): StatPearls Publishing; 2023 Jan-. Available from: https://www.ncbi.nlm.nih.gov/books/NBK563181/
Eddy, E. M., & Shapiro, B. M. (1979). Membrane events of fertilization in the sea urchin. Scanning electron microscopy, (3), 287–297.
Elshazzly M, Lopez MJ, Reddy V, et al. Embryology, Central Nervous System. [Updated 2023 Apr 3]. In: StatPearls [Internet]. Treasure Island (FL): StatPearls Publishing; 2023 Jan-. Available from: https://www.ncbi.nlm.nih.gov/books/NBK526024/
Georgadaki, K., Khoury, N., Spandidos, D. A., & Zoumpourlis, V. (2016). The molecular basis of fertilization (Review). International journal of molecular medicine, 38(4), 979–986. https://doi.org/10.3892/ijmm.2016.2723
Gilbert SF. (2000). Developmental Biology. 6th edition. Sunderland (MA): Sinauer Associates; An Introduction to Early Developmental Processes. Available from: https://www.ncbi.nlm.nih.gov/books/NBK9992/
Hasley, A., Chavez, S., Danilchik, M., Wühr, M., & Pelegri, F. (2017). Vertebrate Embryonic Cleavage Pattern Determination. Advances in experimental medicine and biology, 953, 117–171. https://doi.org/10.1007/978-3-319-46095-6_4
Khan YS, Ackerman KM. Embryology, Week 1. [Updated 2023 Apr 17]. In: StatPearls [Internet]. Treasure Island (FL): StatPearls Publishing; 2023 Jan-. Available from: https://www.ncbi.nlm.nih.gov/books/NBK554562/
Kishimoto T. (2015). Entry into mitosis: a solution to the decades-long enigma of MPF. Chromosoma, 124(4), 417–428.
https://doi.org/10.1007/s00412-015-0508-y
Marlow FL. Maternal Control of Development in Vertebrates: My Mother Made Me Do It! San Rafael (CA): Morgan & Claypool Life Sciences; 2010. Egg Activation. Available from: https://www.ncbi.nlm.nih.gov/books/NBK53183/
National Research Council (US) Subcommittee on Reproductive and Neurodevelopmental Toxicology. Biologic Markers in Reproductive Toxicology. Washington (DC): National Academies Press (US); 1989. 25, Developmental Neurobiology of the Central Nervous System. Available from: https://www.ncbi.nlm.nih.gov/books/NBK218958/
Oliver R, Basit H. Embryology, Fertilization. [Updated 2023 Apr 17]. In: StatPearls [Internet]. Treasure Island (FL): StatPearls Publishing; 2023 Jan-. Available from: https://www.ncbi.nlm.nih.gov/books/NBK542186/
Rao JN, Wang JY. Regulation of Gastrointestinal Mucosal Growth. San Rafael (CA): Morgan & Claypool Life Sciences; 2010. Intestinal Architectuhttps://doi.org/10.1242/dev.200885 https://www.ncbi.nlm.nih.gov/books/NBK54098/
Schmerler, S., & Wessel, G. M. (2011). Polar bodies--more a lack of understanding than a lack of respect. Molecular reproduction and development, 78(1), 3–8. https://doi.org/10.1002/mrd.21266
Serrano Nájera, G., & Weijer, C. J. (2023). The evolution of gastrulation morphologies. Development (Cambridge, England), 150(7), dev200885. https://doi.org/10.1242/dev.200885
Setti, A. S., Figueira, R. C. S., Braga, D. P. A. F., Iaconelli, A., & Borges, E., Jr (2018). Blastomere nucleation: Predictive factors and influence of blastomere with no apparent nuclei on blastocyst development and implantation. JBRA assisted reproduction, 22(2), 102–107. https://doi.org/10.5935/1518-0557.20180028
Solnica-Krezel, L., & Sepich, D. S. (2012). Gastrulation: making and shaping germ layers. Annual review of cell and developmental biology, 28, 687–717. https://doi.org/10.1146/annurev-cellbio-092910-154043
Thiery, J. P., & Sleeman, J. P. (2006). Complex networks orchestrate epithelial-mesenchymal transitions. Nature reviews. Molecular cell biology, 7(2), 131–142. https://doi.org/10.1038/nrm1835
Visual Sources
Figure 1: Sperm Swim Together To Help Each Other Reach The Egg. From: https://www.sciencefriday.com/segments/sperm-swim-together/
Figure 2: Swann, K., & Lai, F. A. (2016). Egg Activation at Fertilization by a Soluble Sperm Protein. Physiological reviews, 96(1), 127–149. https://doi.org/10.1152/physrev.00012.2015
Figure 3: Zygote. From: https://getmegiddy.com/facts-about-zygote
Figure 4: Luckout University. Cleavage patterns of eggs. From: https://www.lkouniv.ac.in/site/writereaddata/siteContent/202004080636590834shailie_Cleavage_types_and_patterns.pdf
Figure 5: Gastrula. From: https://www.lookphotos.com/en/images/70336852-Gastrula
Figure 6: Lumen Learning. Vertebrate Axis Formation. From: https://courses.lumenlearning.com/wm-biology2/chapter/vertebrate-axis-formation/
Figure 7: Fiuza, U. M., & Lemaire, P. (2021). Mechanical and genetic control of ascidian endoderm invagination during gastrulation. Seminars in cell & developmental biology, 120, 108–118. https://doi.org/10.1016/j.semcdb.2021.08.001
Figure 8: Novus Biologicals. Epithelial-Mesenchymal Transition (EMT) Markers. From: https://www.novusbio.com/antibody-news/antibodies/antibodies-for-epithelial-mesenchymal-transition-emt-marker
Figure 9: Embryological Development of the CVS. https://simplemed.co.uk/subjects/cardiovascular/embryology-of-the-cvs.
Figure 10: Development of the Gastrointestinal Tract. From: https://simplemed.co.uk/subjects/gastrointestinal/development-of-the-gastrinstestinal-tract
Figure 11: Neuroendocrine Tumor Research Fundation. Neuroendocrine Tumors of the Gastrointestinal Tract. From: https://netrf.org/old-for-patients/nets-info/tumor-site/gi/
Figure 12: Teach Me Anatomy. Development of the Central Nervous System. 2023. From: https://teachmeanatomy.info/the-basics/embryology/central-nervous-system/
Figure 13: Nutton, Georgina & Silburn, Sven & Arney, Fiona & Moss, Bonnie. (2011). The first five years: Starting early. Early Childhood Series No. 2. Figure 2.
Figure 14: Shutterstock. Brain x-ray image of patient by mri, ct scan for medical diagnosis. From: https://www.shutterstock.com/de/image-photo/brain-xray-image-patient-by-mri-730937659
Comentários