iPSCs and Neurological Diseases: Advancements and Challenges
A new approach dominates the field of cell biology: induced pluripotent stem cells (iPSCs). The study of neurological disorders has always been impeded by challenges such as the accessibility of neuronal tissue. iPSCs are artificially produced by somatic cells and are able to differentiate into all human cell types. Neuronal and brain tissues are now easily accessible, facilitating the study of the underlying mechanisms of neurological disorders and the design of therapeutic strategies against them. Applications of iPSCs have already been conducted in infantile neurological disorders, such as dopamine transporter deficiency syndrome, Dravet syndrome and Spinal Muscular dystrophy (Ng et al., 2021, Birey et al., 2017, Xu, Denton, Wang, Zhang, & Li, 2015). iPSCs are also able to organise into 3D structures, called organoids, illustrating human tissues, such as the brain. iPSCs hold an advantage against animal models, offering much more accuracy about the function of human tissues. However, challenges such as immunogenicity and tumorigenicity need to be overcome before the wide use of iPSCs in biological research and drug design.
How are iPSCs created?
To understand the creation of iPSCs, it is important to know the stages of cell potency in mammalian development. At the embryonic state, the first cell created is the totipotent stem cell. This then develops into the the pluripotent stem cell which has the ability to differentiate into all three types of germ layers; the endoderm line, the mesoderm line and the ectoderm line. These will then become multipotent stem cells and will derive into all discrete cell types, such as neuronal cells, skin cells, blood cells inter alia. (Mahla, 2016). The idea is that by inducing an adult somatic cell into becoming a pluripotent stem cell, it can be reprogrammed to make any tissue. iPSCS were initially described by Professor Yamanaka, who discovered that by reactivating four specific genes in an adult cell, it could return it to the pluripotent state (Yamanaka, 2007). He managed to reprogram adult human somatic cells to generate iPSCs. To achieve that, he used somatic cells to over-express transcription factors important for the maintenance of pluripotency. These are Sox2, Oct4, Klf4 and c-Myc. These transcription factors then activate the silenced genes of pluripotency by demethylating them. After the genes are reactivated, they express transcription factors of pluripotency, enabling mesenchymal to epithelial transition (Takahashi et al., 2007). The iPSCs can now be reprogrammed to the desired cell type.
Human derived iPSCs have facilitated the investigation of the background mechanisms of infantile neurological disorders and have enabled the development of new therapeutic approaches (Figure 2). They can be used as cellular models for in vitro studies or to generate organoids. iPSCs can spontaneously differentiate into the desired cell type. For example, for the production of neurons, the first step is to derive the ectoderm. Blocking the action of TNF and BMP, transcription factors that induce differentiation of mesoderm and endoderm, only ectoderm is produced. Ectodermal cells are then exposed to four other specific transcription factors (SHH, WNT, BDNF, GDNF) to produce basal neural ectoderm cells and finally generate neurons. Consecutive addition of differentiation media can lead to generation of neural organoids (Lancaster et al., 2013) (Figure 2).

Advantages and uses of iPSCs against traditional animal models
iPSCs have facilitated the study of human disease and have provided a wide range of tools promoting drug research. Although animal models have taken the lead for several years now in biological research, advancements in heterologous cellular models have opened a new pathway in scientific research. Animal anatomy often differs from the human one, complicating the understanding of physiological mechanisms. For example, Central Nervous System (CNS) structures, such as the cortex, are distinct between rodents and humans. The human cortex has high cortical folding, presenting convolutions, (gyrencephalic cortex) and contains 16.3 billion neurons. On the contrary, mouse cortex is smooth, not presenting any folding (lissencephalic cortex) and only contains 13.7 millions of neurons. These characteristics are crucial for the cognitive function. Human neuronal complexity and functions are much better depicted by iPSCs and by differentiated neuronal structures. Moreover, considering that animal physiology does not completely resemble the human one, drug testing is much more efficient in heterologous cellular models derived from human cells, as they better depict the toxicology of the human tissue in response to the treatment as well as its tolerability. Clinical trials can now be more efficient, reliable and quick, as treatments can be tested on human tissues before the start of the trial (Desbordes and Studer, 2012, Dolmetsch and Geschwind, 2011). This is beneficial because animal models may have a different reaction to a specific drug than humans. Improvement of gene editing techniques such as CRISPR/Cas9 have made generation of heterologous cellular models quicker and more practical comparing to the generation of mutated mice. Thus, they allow for the study of a particular disease or drug treatment across various genetic backgrounds, incorporating relatively easy gene edits. iPSCs can also be produced in endless amounts and are able to generate cells that are not easily accessible for biopsies (Medvedev, Shevchenko and Zakian, 2010). Regarding the disease profile of a patient, iPSCs enable investigation of the genetic background of a person. iPSCs offer easy access to family history in reference to a particular disease. Nonetheless, the fact that the cells originate from a particular patient, gives the opportunity of personalised treatments and precision medicine (Silva and Haggarty, 2019). Finally, it is important to mention that the bioethical issues that arise from the use of animal models are overcome by the application of iPSCs in biological research.
Patient-derived neurons facilitate research of neurological diseases which, until now, have been mainly investigated in animal models. Accessibility of neuronal tissue has always been a major obstacle in the study of neurological disorders. Patient-derived neurons enable modeling of neuronal diseases and thus better understanding of disease mechanisms. For example, studies of Alzheimer’s disease and amyotrophic lateral sclerosis patients proved that deriving neurons from patients enables investigation of various mutations that may cause a disease and the distinct genetic backgrounds between different phenotypes of the same neuronal disease (Egawa et al., 2012, Tong, Fong & Huang, 2015). This model also enables the discovery of new biomarkers that may not be visible in animals or that may appear in the very early stages of a neuronal disease. Patient-derived neurons serve as a much more reliable model for novel drug discoveries comparing to animal models, as they immediately present toxic and dose response issues of human neuronal tissues. Finally, patient-derived neurons can potentially be used in cell therapies of neuronal diseases. Successful research on Parkinson’s disease (PD) patients, showed that implantation of substantia nigra cells in the patient significantly reduced the neurodegeneration symptoms (Schweitzer et al., 2020). These findings prove that this technology can initiate a new era of personalised medicine for neurodegenerative diseases.
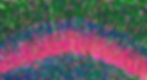
Existing limitations in the use of iPSCs in biological research
Although iPSCs hold a great potential for neurological disease research, there are still a few challenges that need to be overcome before their wide use in the field. iPSCs do not fully mimic the physiology of in vivo human tissue, impeding the complete understanding of cellular mechanisms and the effect of treatments. For now, mouse models are still necessary for illustrating pathophysiological pathways. Moreover, the different genetic backgrounds of patients, lead to the production of genetically different iPSCs.
Heterogeneity between iPSCs is one of the major issues that complicates their utility in neuronal research and drug design. iPSC lines may differ in gene expression, their ability to grow and their structure. The different genetic background is probably the reason of this heterogeneity (Yamanaka, 2020).
Another existing challenge is the modeling of late-onset diseases, sporadic diseases and multifactorial diseases, whose progress entails the involvement of environmental and various other factors. This is probably the result of the incapability of iPSCs to efficiently mature. Such diseases are sporadic Alzheimer’s disease, schizophrenia, and autism spectrum disorders (Doss and Sachinidis, 2019). Obtaining mature cell types is currently easier in animal models, as rigorous research of polygenic and sporadic disorders requires very big amounts of iPSCs (Sterneckert, Reinhardt & Schöler, 2014).
Another concern is the tumorigenicity and immunogenicity of iPSCs. Their ability to proliferate makes them more susceptible to the creation of cancerous cells. Active reprogramming factors may also initiate the formation of tumors. Undifferentiated iPSCS in a population of differentiated ones have been claimed to develop malignant tumors and teratomas during transplantation (Okita, Ichisaka & Yamanaka, 2007). Induction of immune responses after transplantation is also a current issue (Mattapally et al., 2018). Traditionally, immunogenicity issues are overcome by the use of immunosuppressants (Yamanaka, 2020). Although iPSCs can potentially be generated from any kind of somatic cell, it is reported that some cell types, such as skin cells, are more susceptible to mutagenic agents, for example UV light. Therefore, it is important to choose the appropriate cell type to assure safety.

Conclusion
iPSCs offer a wide range of new possibilities in research and drug design of neurological and other diseases. The ability to culture them in vitro, gives accessibility to nearly all human tissues. Scientists can delve deeper into the study of tissues, disease mechanisms and genetics. They also facilitate comparing and contrasting the genetic background and the symptoms of a disease between patients and healthy controls. The evaluation of novel drug treatments in this human-derived system is a great advantage for the development of new therapies, as the treatment is tested on human tissue in the early phases of the research. The currently developing technology of organoids will enable even more meticulous study of human tissue and will open new doors in the applications of cell therapy in neurological diseases. iPSCs seem to slowly replace animal models, at least at the early stages of studies. Challenges in the application of iPSCs, indicate the utility of animal models. The major issues of iPSCs are immunogenicity, heterogeneity and tumorigenicity. However, several experimental techniques are being developed to overcome these limitations.
From a more personal perspective, iPSCs offer a revolutionary platform for disease research and drug design. Their most exciting application is cell therapy, as studies already highlight their ability to heal previously untreatable diseases. Animal models are still crucial for disease and drug research. However, their use in the field and the ethical issues that arise from it, can be eliminated by the dominance of iPSCs. An interdisciplinary approach is required to appreciate the whole potential of iPSCs and organoids in scientific research.
Bibliographical References
Birey, F., Andersen, J., Makinson, C. D., Islam, S., Wei, W., Huber, N., … Paşca, S. P. (2017). Assembly of functionally integrated human forebrain spheroids. Nature, 545(7652), 54–59. doi:10.1038/nature22330 https://doi.org/10.1038/nature22330 Desbordes, S. C., & Studer, L. (2012). Adapting human pluripotent stem cells to high-throughput and high-content screening. Nature Protocols, 8(1), 111–130. doi:10.1038/nprot.2012.139 https://doi.org/10.3389/fphar.2014.00150 Dolmetsch, R., & Geschwind, D. H. (2011). The human brain in a dish: The promise of ipsc-derived neurons. Cell, 145(6), 831–834. doi:10.1016/j.cell.2011.05.034 https://doi.org/10.1016/j.cell.2011.05.034 Doss, M. X., & Sachinidis, A. (2019). Current challenges of IPSC-based disease modeling and therapeutic implications. Cells, 8(5), 403. doi:10.3390/cells8050403
https://doi.org/10.3390/cells8050403 Egawa, N., Kitaoka, S., Tsukita, K., Naitoh, M., Takahashi, K., Yamamoto, T., … Inoue, H. (2012). Drug screening for ALS using patient-specific induced pluripotent stem cells. Science Translational Medicine, 4(145). doi:10.1126/scitranslmed.3004052 https://doi.org/10.1126/scitranslmed.3004052 Lancaster, M. A., Renner, M., Martin, C.-A., Wenzel, D., Bicknell, L. S., Hurles, M. E., … Knoblich, J. A. (2013). Cerebral organoids model human brain development and microcephaly. Nature, 501(7467), 373–379. doi:10.1038/nature12517 https://doi.org/10.1038/nature12517 Mahla, R. S. (2016). Stem cells applications in regenerative medicine and disease therapeutics. International Journal of Cell Biology, 2016, 1–24. doi:10.1155/2016/6940283 https://doi.org/10.1155/2016/6940283 Mattapally, S., Pawlik, K. M., Fast, V. G., Zumaquero, E., Lund, F. E., Randall, T. D., … Zhang, J. (2018). Human leukocyte antigen class I and II knockout human induced pluripotent stem cell–derived cells: Universal donor for cell therapy. Journal of the American Heart Association, 7(23). doi:10.1161/jaha.118.010239
https://doi.org/10.1161/JAHA.118.010239 Medvedev, S. P., Shevchenko, A. I., & Zakian, S. M. (2010). Induced pluripotent stem cells: Problems and advantages when applying them in regenerative medicine. Acta Naturae, 2(2), 18–27. doi:10.32607/20758251-2010-2-2-18-27 https://doi.org/10.32607/20758251-2010-2-2-18-27 Ng, J., Barral, S., De La Fuente Barrigon, C., Lignani, G., Erdem, F. A., Wallings, R., … Kurian, M. A. (2021). Gene therapy restores dopamine transporter expression and ameliorates pathology in ipsc and mouse models of infantile parkinsonism. Science Translational Medicine, 13(594). doi:10.1126/scitranslmed.aaw1564
https://doi.org/10.1126/scitranslmed.aaw1564 Okita, K., Ichisaka, T., & Yamanaka, S. (2007). Generation of germline-competent induced pluripotent stem cells. Nature, 448(7151), 313–317. doi:10.1038/nature05934 https://doi.org/10.1038/nature05934 Schweitzer, J. S., Song, B., Herrington, T. M., Park, T.-Y., Lee, N., Ko, S., … Kim, K.-S. (2020). Personalized ipsc-derived dopamine progenitor cells for parkinson’s disease. New England Journal of Medicine, 382(20), 1926–1932. doi:10.1056/nejmoa1915872 http://dx.doi.org/10.1056/NEJMoa1915872 Silva, M. C., & Haggarty, S. J. (2019). Human pluripotent stem cell–derived models and drug screening in CNS precision medicine. Annals of the New York Academy of Sciences, 1471(1), 18–56. doi:10.1111/nyas.14012 https://doi.org/10.1111/nyas.14012 Sterneckert, J. L., Reinhardt, P., & Schöler, H. R. (2014). Investigating human disease using stem cell models. Nature Reviews Genetics, 15(9), 625–639. doi:10.1038/nrg3764 https://doi.org/10.1038/nrg3764 Takahashi, K., Tanabe, K., Ohnuki, M., Narita, M., Ichisaka, T., Tomoda, K., & Yamanaka, S. (2007). Induction of pluripotent stem cells from adult human fibroblasts by defined factors. Cell, 131(5), 861–872. doi:10.1016/j.cell.2007.11.019 https://doi.org/10.1016/j.cell.2007.11.019 Thomson, J. A., Itskovitz-Eldor, J., Shapiro, S. S., Waknitz, M. A., Swiergiel, J. J., Marshall, V. S., & Jones, J. M. (1998). Embryonic stem cell lines derived from human blastocysts. Science, 282(5391), 1145–1147. doi:10.1126/science.282.5391.1145 https://doi.org/10.1126/science.282.5391.1145 Tong, L. M., Fong, H., & Huang, Y. (2015). Stem cell therapy for alzheimer’s disease and related disorders: Current status and future perspectives. Experimental & Molecular Medicine, 47(3). doi:10.1038/emm.2014.124 https://doi.org/10.1038/emm.2014.124 Xu, C.-C., Denton, K. R., Wang, Z.-B., Zhang, X., & Li, X.-J. (2015). Abnormal mitochondrial transport and morphology as early pathological changes in human models of spinal muscular atrophy. Disease Models & Mechanisms. doi:10.1242/dmm.021766 https://doi.org/10.1242/dmm.021766 Yamanaka, S. (2007). Strategies and new developments in the generation of patient-specific pluripotent stem cells. Cell Stem Cell, 1(1), 39–49. doi:10.1016/j.stem.2007.05.012
Visual Sources
Figure 1: Barral Serena & Kurian Manju (2016), Use of patient-derived induced pluripotent stem cells (iPSCs) for modeling genetic neurological diseases (Diagram), Frontiers, https://www.frontiersin.org/articles/10.3389/fnmol.2016.00078/full Figure 2: Neurons are not the only brain cells that think (2023). [Image]. The Economist https://www.economist.com/science-and-technology/2023/01/23/neurons-are-not-the-only-brain-cells-that-think
Figure 3: Shinya Yamanaka MD, PhD (2012) (Photograph) University of California San Francisco, https://www.ucsf.edu/news/2012/10/104393/shinya-yamanaka-wins-2012-nobel-prize-medicine