Glucocorticoids: the Most Effective Class of Anti-Inflammatory Medications
Our body consists of a complex network of cells, tissues, and organs that work together to defend us against harmful substances, such as bacteria, viruses, fungi, and other pathogens. This intricate network is collectively referred to as the immune system, which plays a crucial role in defending our body from infections and diseases. When the immune system detects a threat, it promptly initiates a cascade of biochemical and cellular processes with the purpose of eliminating the injurious agent and, consequently, leading to the manifestation of classic signs of inflammation. These include rubor (redness), calor (warmth), tumor (swelling), dolor (pain), and functio laesa (loss of function). Although inflammation often causes unpleasant sensations, it is a beneficial and protective response to illness, injury, or infection, that needs to be finely regulated. In certain circumstances, such as autoimmune diseases, the immune system mistakenly attacks the body's own tissues and immunosuppressive medicines may be prescribed to reduce the immune response and decrease inflammation.
Glucocorticoids (GCs) are potent anti-inflammatory and immunosuppressive agents that are widely used in clinical practice for the treatment of various inflammatory and autoimmune conditions. The ability of natural GCs to suppress a variety of pro-inflammatory molecules and to induce certain anti-inflammatory molecules has been greatly explored with the use of synthetic GCs (artificial) for the treatment of numerous diseases. Synthetic GCs are artificial molecules that resemble natural GCs. GCs are critical regulators of inflammatory and immune responses, as well as a wide variety of fundamental physiological processes, including metabolism and cell proliferation, development and reproduction (Vandevyver, Dejager et al. 2013). Imbalances in GC levels can lead to pathological conditions such as Cushing's disease, characterized by persistent elevation of GCs, and Addison's disease, which involves GC deficiency.
Natural GCs, such as cortisol in humans, are hormones derived from cholesterol and are produced and secreted by the adrenal glands (also known as suprarenal glands, located on top of both kidneys and responsible for producing numerous hormones). The synthesis and release of these GCs in the body is tightly regulated by a complex system (discussed below), and their production follows a dynamic circadian rhythm (the body's internal clock that follows a 24-hour cycle and regulates various bodily functions such as sleep patterns). In humans, GCs are mainly released in the early morning hours (Figure 1).
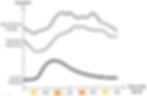
Background and applications
The discovery of GCs happened when the physician Thomas Addison initially characterized Addison's illness (later referred to as adrenal insufficiency, which is when the adrenal glands don't produce enough hormones) in the 19th century. Thomas Addison made a significant observation that the administration of adrenal gland extracts could be beneficial to patients suffering from weight loss, chronic fatigue, muscular degeneration, and darkening of the skin, all features of Addison's disease. In 1946, Edward Calvin Kendall isolated the compounds A, B, E, and F, from adrenal extracts. Shortly after, rheumatologist Philip Hench first recognized the GCs' medicinal potential in a patient with rheumatoid arthritis who responded favourably to compound E. Later, compound E would become known as cortisol and was synthesized in 1946 by Sarett. Hench, Kendall and Tadeus Reichstein, who succeeded in isolating several steroid hormones from the adrenal glands eventually leading to the discovery of cortisol, were awarded the Nobel Prize for Medicine and Physiology in 1950 (Timmermans, Souffriau et al. 2019).
Since then, GCs have revolutionized the medicine practice, becoming part of the group of most used and cost-effective anti-inflammatory medications. At therapeutic concentrations, GCs have potent anti-inflammatory effects, and thus many diseases or disorders of an autoimmune, inflammatory or allergic nature are often treated with synthetic GCs such as dexamethasone or prednisolone (Vandevyver, Dejager et al. 2013). GCs are also prescribed as immunosuppressants (when the immune system is intentionally weakened or suppressed) for transplant patients, in addition to acting in the treatment of cancer, due to their anti-proliferative and anti-angiogenic actions, properties associated with cancer proliferation and growth (Kadmiel and Cidlowski 2013). The most recent example of GC therapy was during the COVID-19 pandemic (Bruscoli, Puzzovio et al. 2022). GCs were recommended by various regulatory agencies for the treatment of COVID-19 patients requiring oxygen therapy. However, the use of GCs to treat COVID-19 is a topic of ongoing debate. Factors such as the timing and duration of treatment, the phase of the disease, and the presence of co-morbidities (presence of two or more medical conditions) can significantly impact the effectiveness and potential side effects of GCs (Bruscoli, Puzzovio et al. 2022). As a result, numerous clinical studies are currently being conducted to better understand the role of GCs in COVID-19 treatment.
Natural GCs (such as cortisol in humans) play a critical role in controlling the inflammatory process. As previously mentioned, cortisol is a hormone produced and secreted by the adrenal gland, and its circulating concentration is regulated through the interaction between the hypothalamus, pituitary gland, and adrenal glands, called the hypothalamic-pituitary-adrenal (HPA) axis (Figure 2), (Kadmiel and Cidlowski 2013). During the inflammatory process, pro-inflammatory cytokines stimulate the production of Corticotropin-Releasing Hormone (CRH), which, consequently, triggers the release of Adrenocorticotropic Hormone (ACTH) by the pituitary gland. ACTH, in turn, induces the synthesis of cortisol by the cells in the fasciculata zone of the adrenal gland cortex (Figure 2) (Gross, Lu et al. 2009, Silverman and Sternberg 2012). In addition to systemic production, there is experimental evidence of local production of corticosteroids observed in different tissues, including primary lymphoid organs, the intestine, the skin, the brain, and possibly the heart (Taves, Gomez-Sanchez et al. 2011).

Glucocorticoid receptors
A receptor is a molecule inside or on the surface of a cell that binds to a specific substance and causes a specific effect in the cell. Both the physiological and pharmacological actions of GCs are mediated by the glucocorticoid receptor (GR)(Oakley and Cidlowski 2013). GRs are constitutively expressed cytoplasmic proteins that act by altering the expression of target genes (segments of DNA that act as instructions for making molecules) in the nucleus of the cell, in response to a specific hormonal signal.
In recent years, the traditional concept that GCs act through a single type of GR has been modified with the discovery of a wide variety of receptor subtypes (Oakley and Cidlowski 2013). Among the isoforms, GRα and GRβ are isoforms of the human GR that have been identified. GRα is the predominant isoform, associated with binding to GCs and activation of target genes in the nucleus. GRβ is not responsive to GCs and differs from GRα in its structure (Oakley and Cidlowski 2011, Ligr, Li et al. 2012). Therefore, the binding of GCs to GRβ does not generate any gene activation. GCs resistance (discussed below) has been partly attributed to higher levels of the β-isoform in some patients (Barnes 2011).
Mechanism of action
Due to their high lipophilicity (the ability of a molecule to dissolve in or interact with lipids or fats), GCs are capable of crossing the cytoplasmic membrane of the cell via passive transport. In the absence of cortisol or synthetic GC binding, the glucocorticoid receptor (GR) remains inactive in the cytoplasm, stabilized by a protein complex known as heat shock proteins (HSPs) and other factors, forming a heterocomplex, that prevents its degradation and assists in its maturation (Vandevyver, Dejager et al. 2013).
Once in the cytoplasm of the target cell, GCs can bind to GRs, leading to a conformational change that results in the dissociation of the heterocomplex with stabilizing proteins. This leads to the exposure of a specific segment, the nuclear localization signal, allowing the GC-GR homodimer to translocate to the nucleus (Oakley and Cidlowski 2011). In the nucleus, the GC-GR complex binds to specific sites on the DNA called glucocorticoid response elements (GREs). This results in the induction of anti-inflammatory protein synthesis (such as annexin A1, glucocorticoid-induced leucine zipper, IκB, and IL-10) and the regulation of proteins involved in systemic metabolism or the regulation of pro-inflammatory genes (Clark 2007, Vandevyver, Dejager et al. 2013). This process is called transactivation, and most of the adverse effects (undesirable outcomes) associated with GCs appear to be related to this mechanism.
GCs also act through another mechanism called transrepression, in which GC molecules and GC receptors interact with molecules involved in the regulation of pro-inflammatory genes, such as NF-κB and AP-1. The inhibition of these molecules leads to the suppression of the synthesis of pro-inflammatory mediators, including cytokines, and chemokines, among others (Clark 2007, Busillo and Cidlowski 2013). The mechanisms of transactivation and transrepression are represented in Figure 3.

Adverse side effects and resistance
While GCs possess excellent anti-inflammatory efficacy, their therapeutic use requires careful control. One significant challenge is the development of tissue resistance in patients undergoing chronic GC therapy, which substantially limits the effectiveness of the treatment. This resistance means that over time, the desired response to GCs diminishes, making it more difficult to achieve the desired therapeutic outcomes. It is important for healthcare professionals to closely monitor patients receiving long-term GC therapy and adjust the treatment plan whenever necessary. Many inflammatory diseases, such as chronic obstructive pulmonary disease, pulmonary fibrosis, and cystic fibrosis, are largely resistant to GC treatment. In addition to therapy resistance, the use of GCs is often accompanied by severe adverse effects, including metabolic syndrome, bone-density loss, diabetes, obesity, cardiovascular diseases, and psychiatric symptoms, as represented in Figure 4 (Barnes 2011, Oakley and Cidlowski 2011, Vandevyver, Dejager et al. 2013). Additionally, GCs can increase susceptibility to infections due to immunosuppression (which is when the immune system is weakened or suppressed by the use of GCs). Since prolonged use of GCs leads to suppression of the hypothalamic-pituitary-adrenal axis (HPA) inhibiting the natural production of cortisol, it is not recommended to abruptly discontinue treatment so the spontaneous production can return (Clark 2007, Beaulieu and Morand 2011).

The adverse metabolic effects of GCs seem to be dependent on the induction of gene expression, ie, transactivation (Vandevyver, Dejager et al. 2013). Consequently, so-called selective GR agonists (SEGRAs), which favour transrepression (inhibition of pro-inflammatory molecules) over transactivation, have been developed as therapeutic agents to reduce side effects, but so far it has been difficult to dissociate anti-inflammatory effects from adverse effects. Furthermore, some studies indicate that GR-induced transactivation is essential for GCs' anti-inflammatory properties, and this strategy to improve therapy with GCs has not been successful (Vandevyver et al., 2013). In patients with GC resistance, medicines that may reverse the molecular mechanisms of GC resistance are being investigated, as well as alternative anti-inflammatory treatments (Barnes 2011).
Conclusions
For decades, GCs have been used to treat various inflammatory conditions. Their efficacy stems from their ability to effectively inhibit the production of pro-inflammatory mediators and reduce inflammation. This class of anti-inflammatory medicines is commonly used in clinical practice and represents a pioneering achievement in harnessing the therapeutic potential of an endogenous molecule, cortisol. Although GCs are widely used to control inflammation in various diseases, it is crucial to monitor their use by specialists to ensure a more effective, safe therapy, and better quality of life for the patient. Currently, there are several research groups committed to improving the therapeutic approach of these medicines, aiming to reduce the associated adverse effects and resistance. While the primary mechanisms of action GCs are already known, additional studies are necessary to deepen our understanding of these mechanisms. This can not only be beneficial in avoiding undesirable effects but also in further enhancing the therapeutic efficacy of this class of medications.
References
Barnes, P. J. (2011). "Glucocorticosteroids: current and future directions." Br J Pharmacol 163(1): 29-43.
Beaulieu, E. and E. F. Morand (2011). "Role of GILZ in immune regulation, glucocorticoid actions and rheumatoid arthritis." Nat Rev Rheumatol 7(6): 340-348.
Bruscoli, S., P. G. Puzzovio, M. Zaimi, K. Tiligada, F. Levi-Schaffer and C. Riccardi (2022). "Glucocorticoids and COVID-19." Pharmacol Res 185: 106511.
Busillo, J. M. and J. A. Cidlowski (2013). "The five Rs of glucocorticoid action during inflammation: ready, reinforce, repress, resolve, and restore." Trends Endocrinol Metab 24(3): 109-119.
Clark, A. R. (2007). "Anti-inflammatory functions of glucocorticoid-induced genes." Mol Cell Endocrinol 275(1-2): 79-97.
Cooper, M. S., H. Zhou and M. J. Seibel (2012). "Selective glucocorticoid receptor agonists: glucocorticoid therapy with no regrets?" J Bone Miner Res 27(11): 2238-2241.
Gross, K. L., N. Z. Lu and J. A. Cidlowski (2009). "Molecular mechanisms regulating glucocorticoid sensitivity and resistance." Mol Cell Endocrinol 300(1-2): 7-16.
Hoes, J. N., J. W. Jacobs, F. Buttgereit and J. W. Bijlsma (2010). "Current view of glucocorticoid co-therapy with DMARDs in rheumatoid arthritis." Nat Rev Rheumatol 6(12): 693-702.
Kadmiel, M. and J. A. Cidlowski (2013). "Glucocorticoid receptor signaling in health and disease." Trends Pharmacol Sci 34(9): 518-530.
Ligr, M., Y. Li, S. K. Logan, S. Taneja, J. Melamed, H. Lepor, M. J. Garabedian and P. Lee (2012). "Mifepristone inhibits GRbeta coupled prostate cancer cell proliferation." J Urol 188(3): 981-988.
Mohd Azmi, N. A. S., N. Juliana, S. Azmani, N. Mohd Effendy, I. F. Abu, N. I. Mohd Fahmi Teng and S. Das (2021). "Cortisol on Circadian Rhythm and Its Effect on Cardiovascular System." Int J Environ Res Public Health 18(2).
Oakley, R. H. and J. A. Cidlowski (2011). "Cellular processing of the glucocorticoid receptor gene and protein: new mechanisms for generating tissue-specific actions of glucocorticoids." J Biol Chem 286(5): 3177-3184.
Oakley, R. H. and J. A. Cidlowski (2013). "The biology of the glucocorticoid receptor: new signaling mechanisms in health and disease." J Allergy Clin Immunol 132(5): 1033-1044.
Silverman, M. N. and E. M. Sternberg (2012). "Glucocorticoid regulation of inflammation and its functional correlates: from HPA axis to glucocorticoid receptor dysfunction." Ann N Y Acad Sci 1261: 55-63.
Taves, M. D., C. E. Gomez-Sanchez and K. K. Soma (2011). "Extra-adrenal glucocorticoids and mineralocorticoids: evidence for local synthesis, regulation, and function." Am J Physiol Endocrinol Metab 301(1): E11-24.
Timmermans, S., J. Souffriau and C. Libert (2019). "A General Introduction to Glucocorticoid Biology." Front Immunol 10: 1545.
Vandevyver, S., L. Dejager, J. Tuckermann and C. Libert (2013). "New insights into the anti-inflammatory mechanisms of glucocorticoids: an emerging role for glucocorticoid-receptor-mediated transactivation." Endocrinology 154(3): 993-1007.