The Physical Brain: How Neurons Communicate
The nervous system is the most complex system in the human body, if not in biology all together. 86 billion cells make up this system, which is responsible for everything a person perceives, thinks and even feels (Azevedo et al., 2009). As more research is done on the human brain and cognitive behaviour, it seems increasingly evident that everything about a human is physical, and even the most complex emotions are caused by nothing but chemicals. In this article, some light will be shed on how the physical transduction of signals makes up an enormously powerful network in the human nervous system, and what happens when this network malfunctions.
Neurons
The number of neurons in the human body is remarkable. By letting in (influx) and out (efflux) charged particles, signals are transduced between countless cells in the brain. All neurons are capable of capturing and transducing signals. Like all other cells, a neuron has a cell body, called the soma. Various cellular processes that would happen in a typical cell body also take place in the neuronal soma (Wilson & Groves, 1980). The nucleus harbours the genome, mitochondria produce energy-rich molecules, and much more. Unique to neurons, however, are dendrites and axons. Dendrites are long arms of neuronal cells that take in signals and process them. Like many other features in molecular biology, these signals can be either inhibitory or stimulatory (Lovinger, 2008).
Axons are arms used for further signal transduction. In other words, axons produce the output of a neuronal cell, whereas dendrites register the input. An axon also splits into many different branches, each of which delivers the signal to a target cell. More interestingly, axons contain an outer layer known as a myelin sheath, which enables incredibly fast travel of ions (Lovinger, 2008).

Neuronal Communication
Communication between neurons takes place at a synapse. This is the name for the junction between an axon and a dendrite, where information flows from the first cell, or the presynaptic cell, via its axon to the dendrite of the second cell, or the postsynaptic cell. These signals are transduced through molecules called neurotransmitters (Lovinger, 2008).
Within a neuron, a signal is transduced via electrical pulses (Lovinger, 2008). Charged atoms or molecules, called ions, are let in or out of a neuron by cellular proteins. This results in a voltage difference between the cell and the environment outside of the cell, which allows electrical pulses to be generated. Because different neurons are not attached to one another by continuous physical filaments like dendrites and axons, electrical pulses cannot reach other neurons (Grider et al., 2022). Therefore, the presynaptic and postsynaptic cells communicate through the secretion of communication molecules, neurotransmitters, at the synapse. In other words, intracellular communication results from electricity whereas intercellular communication is achieved by neurotransmitters.
Electrical signals that a neuron transduces are a consequence of voltage differences between the environment outside the cell (extracellular) and within the cell (intracellular), separated by the cell membrane. Many different kinds of ions are present across the human body, and neurons take them up very selectively (Grider et al., 2022). As a result, the voltage difference between the extra- and intracellular environment is dynamic and can change by the cell’s opening of ion channels. The two main channels involved are the sodium and potassium channels (Grider et al., 2022).
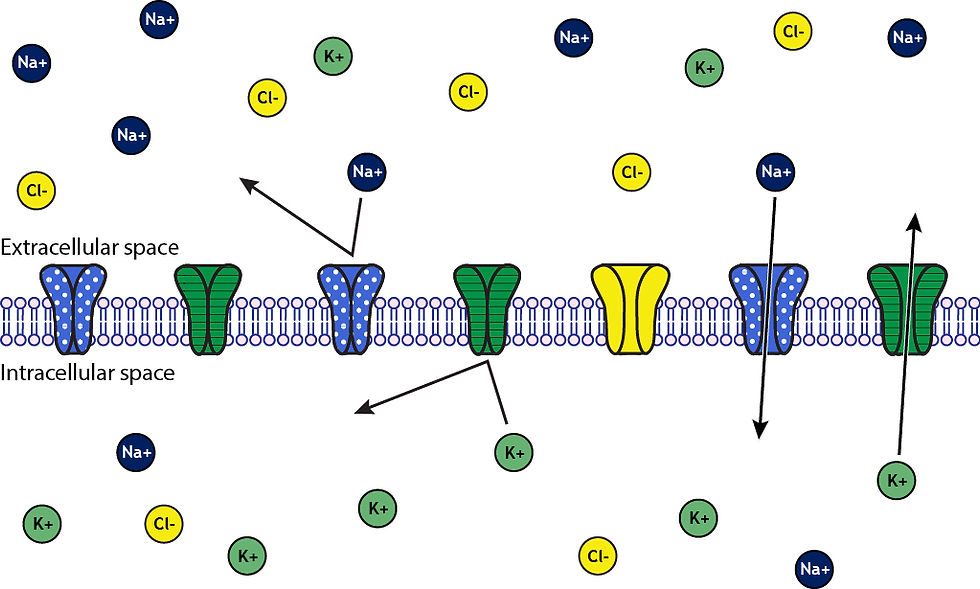
When a neuron is not stimulated, it is said to be at resting potential (Grider et al., 2022). Relatively speaking, the intracellular environment is more negatively charged than the extracellular environment, giving rise to a negative resting potential (Grider et al., 2022). When a neuron becomes excited, it undergoes depolarisation. In this process, sodium channels open, which allows for the influx of positively charged sodium (Na+) and the efflux of positively charged potassium (K+). However, as the sodium flow is greater than that of potassium, the potential grows and eventually becomes positive (Grider et al., 2022). After this excitation potential, potassium channels open to enable influx of potassium and efflux of sodium in the same ratio as before. As a consequence, the cell falls back into its resting potential.
Myelin forms a layer on axons and stops ions from flowing in and out of the cell. As a result, myelinated axons can transmit electrical pulses incredibly rapidly up to 120 metres per second (Siegel & Sapru, 2006). Axons consist of segments, each of which is myelinated. The gap between each segment is called a node of Ranvier (Susuki, 2013). These nodes are uninsulated and contain large numbers of ion channels, similar to those in dendrites. When an impulse reaches a node of Ranvier, the ion channels quickly open due to voltage difference, causing depolarisation as the transfer of ions takes place (Susuki, 2013). As depolarisation only occurs at nodes of Ranvier but not along myelinated segments, signals can travel more rapidly as they jump from one node to another. This way of signal transduction is termed saltatory conduction (Purves et al., 2001). Dendrites, on the other hand, are not coated with a layer of myelin, meaning that there is no insulating layer surrounding dendrites to prevent ion flow. Local depolarisation thus needs to take place more often for a signal to travel down a dendrite, which results in slower transmission speeds compared to axons (Grider et al., 2022). Nonetheless, because dendrites are typically shorter than axons, there is no need for signals to travel as fast (Kemal et al., 2022).
Generally speaking, neurons are not in direct contact with each other (Lovinger, 2008). Instead, they communicate with each other through the synapse. At the axon end, neurons have vesicles with neurotransmitters. When an action potential reaches the neuron, the vesicles open and release neurotransmitters into the synapse, affecting the postsynaptic cell. There are many different kinds of neurotransmitters, and each will bind its specific receptor at the postsynaptic cell. This binding leads to inhibition or excitation of signals through the other cells, once again by an influx or efflux of sodium ions (Lovinger, 2008).
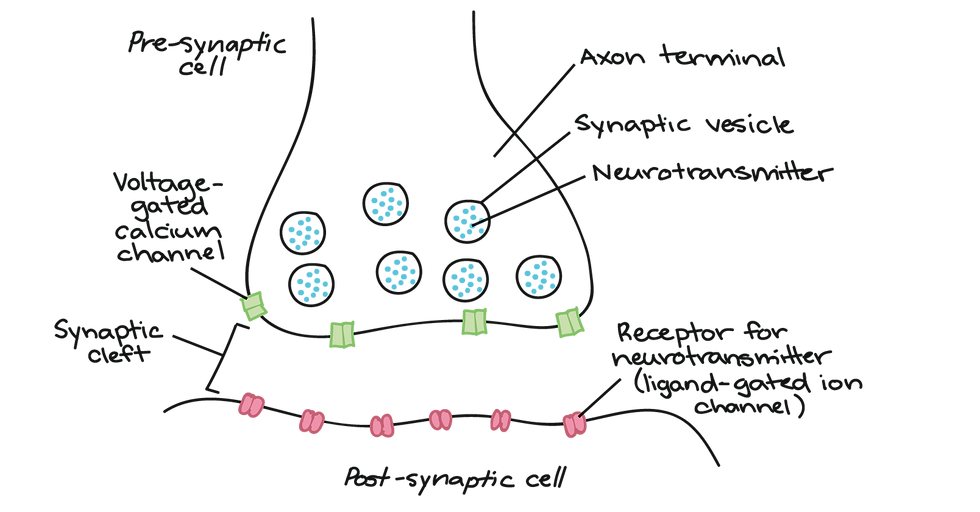
Each neurotransmitter has its own course of action. Dopamine is one of the most well-known neurotransmitters in the human body. It is involved in brain reward mechanisms, and so it has been linked to pleasurable feelings (D’Aquila et al., 2000; Girault & Greengard, 2004; Lovinger, 2008). While its mechanism of action is complicated, an important feature of dopamine is its usage as an antidepressant. There are five different receptors for dopamine and each works in its own way (Lovinger, 2008). In other words, different cells respond variably to the same chemical. The consequence of a neurotransmitter depends on its localisation, as well as which cell it targets (Levinger, 2008). This feature makes for an incredibly complex network.
Neurons in a Network
The network that billions of neurons make up is incredibly complex. An immense amount of research has been done over the past decades to uncover the secrets of neurons and the brain, but much remains unknown. The brain and the nervous system are involved in sending and registering signals to and from all over the body. For instance, one of the most important responsibilities of the brain is its automatic regulation of breathing, among other functions (Guz, 1997).
Apart from physical properties of the body, the brain is also involved in governing cognitive processes. Emotions are effectively nothing more than billions of cells and molecules interacting with one another. These interactions can be based on internal and external stimuli, genetics, and past experiences. They eventually lead to complex signaling cascades in neurons that result in an emotional state of mind. For example, happiness is linked to the secretion of serotonin, oxytocin, and epinephrine, among other chemicals. These neurotransmitters and hormones influence how, and which, neurons communicate with each other (Vyshnavi V Rao & ShamsiyaRizwana, 2020).
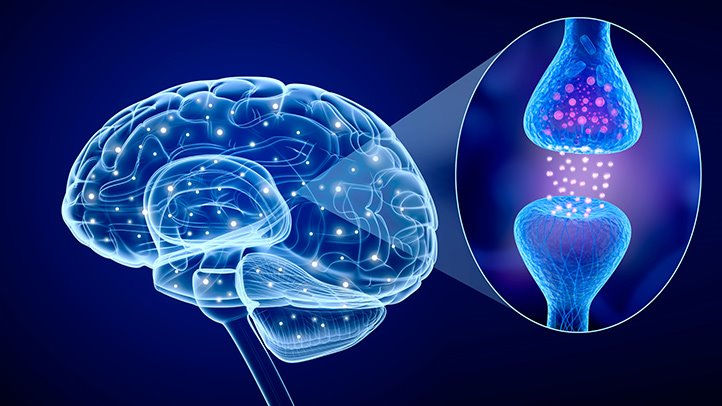
Owing to the complexity of the neuronal network, results can be catastrophic when a part of the system malfunctions. This can be seen in various diseases or disorders related to the nervous system and the brain. Parkinson’s Disease is the second most common neurodegenerative disorder, after Alzheimer’s Disease (Dexter & Jenner, 2013). In patients with Parkinson’s, dopamine levels are substantially lower than that in healthy individuals, as a result of brain cells in a specific region dying (Dexter & Jenner, 2013). This causes a large variety of symptoms, including tremors, posture changes, and depression.
Physicalism and the Brain
As increasing neuroscientific research is available, scientists have come up with physical explanations of things that humans have once thought of as non-physical. Research has shown, for example, that brain damage is linked to an increased likelihood of criminal behaviour (Marr, 2020; Williams et al., 2018). The philosophical stream suggesting that everything in the universe is physical is called physicalism (Wilson, 2006). Combining the concept of physicalism and modern neuroscience, it can be argued that every emotion, personality, and thought running through a human brain at any time is a result of physical molecular interactions and chemical processes taking place in the brain. However, given that the current understanding of physics and neuroscience is most likely incomplete, the science to fully comprehend all these interactions remains unavailable.
That being said, the implications of this hypothesis are enormous in terms of futuristic science. One such possibility is mind-uploading. If physicalism is indeed true, it means that any personality of an individual can be extracted based on the location of each particle inside the human body. If computers with the required power are ever made, then in theory one could digitalise the information of all these particles and practically upload an entire mind (Agar, 2012). Currently, though, neuroscience is nowhere near unravelling individual thoughts and cognitive processes based on the positions of their atoms.
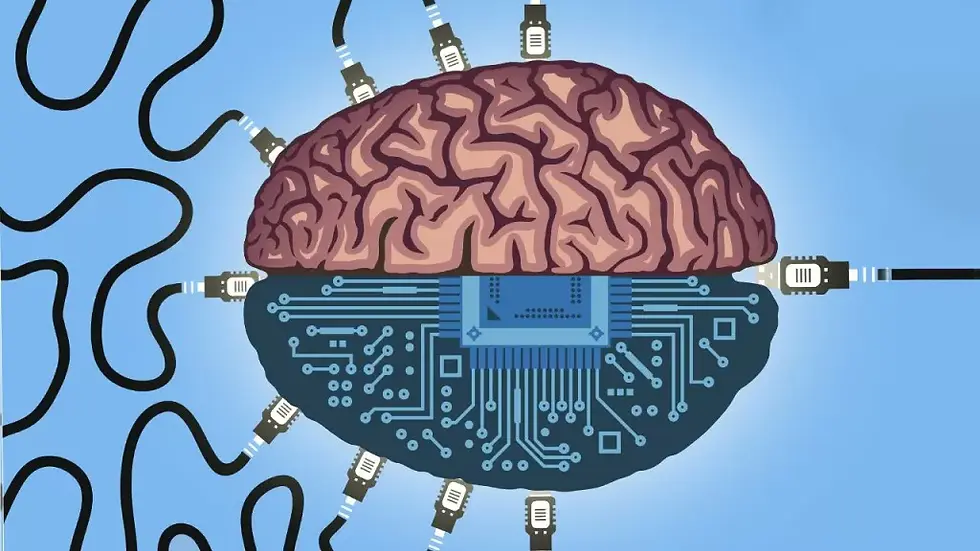
The influence of the nervous system on the human body has yet to be fully understood. The way that a signal is fed through a single neuron itself is amazingly complex, not to mention the communication between neurons using neurotransmitters and receptors. The fact that our nervous system contains billions of cells functioning together to form networks that govern our thoughts and behaviour constitutes it as one of the most complicated systems in biology. Perhaps more people should appreciate the beautiful complexity of the human brain, and its mind.
Bibliographical references
Agar, N. (2012). On the irrationality of mind-uploading: a rely to Neil Levy. AI & SOCIETY, 27(4), 431–436. https://doi.org/10.1007/s00146-011-0333-7
Azevedo, F. A. C., Carvalho, L. R. B., Grinberg, L. T., Farfel, J. M., Ferretti, R. E. L., Leite, R. E. P., Filho, W. J., Lent, R., & Herculano-Houzel, S. (2009). Equal numbers of neuronal and nonneuronal cells make the human brain an isometrically scaled-up primate brain. The Journal of Comparative Neurology, 513(5), 532–541. https://doi.org/10.1002/cne.21974
D’Aquila, P. S., Collu, M., Gessa, G. L., & Serra, G. (2000). The role of dopamine in the mechanism of action of antidepressant drugs. European Journal of Pharmacology, 405(1–3), 365–373. https://doi.org/10.1016/S0014-2999(00)00566-5
Dexter, D. T., & Jenner, P. (2013). Parkinson disease: from pathology to molecular disease mechanisms. Free Radical Biology and Medicine, 62, 132–144. https://doi.org/10.1016/j.freeradbiomed.2013.01.018
Girault, J.-A., & Greengard, P. (2004). The neurobiology of dopamine signaling. Archives of Neurology, 61(5), 641. https://doi.org/10.1001/archneur.61.5.641
Grider, M. H., Jessu, R., & Kabir, R. (2022). Physiology, Action Potential. In StatPearls. StatPearls Publishing.
Guz, A. (1997). Brain, breathing and breathlessness. Respiration Physiology, 109(3), 197–204. https://doi.org/10.1016/S0034-5687(97)00050-9
Kemal, S., Richardson, H. S., Dyne, E. D., & Fu, M. (2022). ER and Golgi trafficking in axons, dendrites, and glial processes. Current Opinion in Cell Biology, 78, 102119. https://doi.org/10.1016/j.ceb.2022.102119
Lovinger, D. M. (2008). Communication networks in the brain: neurons, receptors, neurotransmitters, and alcohol. Alcohol Research & Health : The Journal of the National Institute on Alcohol Abuse and Alcoholism, 31(3), 196–214. http://www.ncbi.nlm.nih.gov/pubmed/23584863
Marr, C. (2020). Neurological abnormalities’ impact on crime and behavior. UTSA Journal of Undergraduate Research and Scholarly Works, 7, 1–16.
Purves, D., Augustine, G. J., Fitzpatrick, D. , Lawrence, K. C., LaMantia, A., McNamara, J. O., & Williams, M. (2001). Neuroscience (2nd ed.). Sunderland, Massachusetts: Sinauer Associates.
Siegel, A., & Sapru, H. , N. (2006). Essential Neuroscience (2nd ed.). Philadelphia : Lippincott Williams & Wilkins.
Susuki, K. (2013). Node of Ranvier disruption as a cause of neurological diseases. ASN Neuro, 5(3), 209–219. https://doi.org/10.1042/AN20130025
Vyshnavi, V. Rao, & ShamsiyaRizwana, V. N. M. B. (2020). Chemistry of emotions - a review. International Journal for Modern Trends in Science and Technology, 6(10), 26–30. https://doi.org/10.46501/IJMTST061005
Williams, W. H., Chitsabesan, P., Fazel, S., McMillan, T., Hughes, N., Parsonage, M., & Tonks, J. (2018). Traumatic brain injury: a potential cause of violent crime? The Lancet Psychiatry, 5(10), 836–844. https://doi.org/10.1016/S2215-0366(18)30062-2
Wilson, C. J., & Groves, P. M. (1980). Fine structure and synaptic connections of the common spiny neuron of the rat neostriatum: A study employing intracellular injection of horseradish peroxidase. The Journal of Comparative Neurology, 194(3), 599–615. https://doi.org/10.1002/cne.901940308
Wilson, J. (2006). On characterizing the physical. Philosophical Studies, 131(1), 61–99. https://doi.org/10.1007/s11098-006-5984-8
Visual Sources
Figure 1: Boeree, C., G. (2009). The Neuron. [image]
http://webspace.ship.edu/cgboer/theneuron.htm
Figure 2: Henley, C. (2021). Foundations of Neuroscience Open Edition. East Lansing: Michigan State University. P368. [image]
Figure 3: Khanacademy. (n.d.). The Synapse. [image]
https://www.khanacademy.org/science/biology/human-biology/neuron-nervous-system/a/the-synapse
Figure 4: Amin, N. (2018). Pharmacological Manipulation of a Brain-Signaling Mechanism Reverses an Autism-Related Pathway. [image] https://www.labroots.com/trending/drug-discovery-and-development/12958/pharmacological-manipulation-brain-signaling-mechanism-reverses-autism-related-pathway
Figure 5: NDZ. (n.d.). Which Is Better a Computer? Or a Human Brain?
https://www.ndimensionz.com/2016/03/24/which-is-better-a-computer-or-a-human-brain/
Cover Image: Pappas, S. (2019). Electrical Stimulation Makes Old Brains Act Young Again. [image] https://www.livescience.com/65177-electrical-stimulation-brain-memory.html
Comments